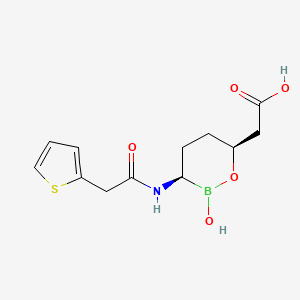
Vaborbactam
Overview
Description
Vaborbactam (formerly RPX7009) is a non-β-lactam β-lactamase inhibitor (BLI) belonging to the cyclic boronic acid pharmacophore class. It is co-administered with the carbapenem antibiotic meropenem to restore its activity against carbapenem-resistant Enterobacteriaceae (CRE), particularly Klebsiella pneumoniae carbapenemase (KPC)-producing strains . This compound inhibits Ambler class A and C β-lactamases, including KPC enzymes, by forming a reversible covalent bond with the active-site serine residue, mimicking the tetrahedral transition state of β-lactam hydrolysis .
Biochemical Analysis
Biochemical Properties
Vaborbactam is a boronic acid β-lactamase inhibitor with a high affinity for serine β-lactamases, including Klebsiella pneumoniae carbapenemase (KPC) . This compound inhibits a variety of β-lactamases, exhibiting a 69 nM K_i against the KPC-2 carbapenemase . It functions as transition state analogs of serine carbapenemase-catalyzed lactam hydrolysis and thereby inhibit these enzymes .
Cellular Effects
This compound restores potency to existing antibiotics by inhibiting the β-lactamase enzymes that would otherwise degrade them . When combined with an appropriate antibiotic, it can be used for the treatment of gram-negative bacterial infections .
Molecular Mechanism
This compound’s mechanism of action involves inhibiting the β-lactamase enzymes that would otherwise degrade antibiotics . It forms reversible covalent bonds with alcohols such as the active site serine in a serine carbapenemase . This property enables them to function as transition state analogs of serine carbapenemase-catalyzed lactam hydrolysis and thereby inhibit these enzymes .
Temporal Effects in Laboratory Settings
The pharmacokinetics of this compound have been evaluated in a neutropenic mouse thigh infection model, as well as in an in vitro hollow-fiber infection model . This compound dosage regimens were designed to produce a wide range of 24-h areas under the concentration-time curves (AUCs) in the thigh infection model .
Dosage Effects in Animal Models
In both the animal and in vitro models, the PK-PD parameter that best described the antibacterial activity of this compound, when administered in combination with meropenem at exposures equivalent to 2 g dosed q8h by 3-h infusion in humans, was the 24-h free this compound AUC/meropenem-vaborbactam (with this compound at 8 mg/liter) MIC ratio .
Metabolic Pathways
This compound does not undergo metabolism . It predominantly undergoes renal excretion, where about 75 to 95% of the dose is excreted unchanged in the urine over a 24 to 48 hour period .
Transport and Distribution
This compound is administered intravenously . It is predominantly excreted through the kidneys .
Subcellular Localization
As a β-lactamase inhibitor, this compound operates in the periplasmic space of gram-negative bacteria where it inhibits β-lactamase enzymes .
Biological Activity
Vaborbactam is a cyclic boronic acid β-lactamase inhibitor developed to combat antibiotic resistance, particularly in carbapenem-resistant Enterobacteriaceae (CRE). Its primary role is to restore the efficacy of carbapenems, such as meropenem, against bacteria that produce class A and C β-lactamases. This article delves into the biological activity of this compound, highlighting its mechanism of action, clinical efficacy, and relevant case studies.
This compound inhibits β-lactamases, which are enzymes produced by bacteria that confer resistance to β-lactam antibiotics. Specifically, it targets:
- Ambler Class A β-lactamases : Including KPC (Klebsiella pneumoniae carbapenemase).
- Class C β-lactamases : Such as those produced by Enterobacter species.
However, this compound shows limited activity against class B metallo-β-lactamases (MBLs) and class D carbapenemases (e.g., OXA-48) .
In Vitro Activity
The effectiveness of this compound has been assessed through various studies:
- Inhibition Potency : this compound demonstrated potent inhibitory activity with minimum inhibitory concentrations (MICs) significantly lower when tested in combination with carbapenems compared to other β-lactams. For example, the MIC for meropenem against KPC-producing strains was reduced from 64 μg/ml to lower levels when combined with this compound .
Drug Combination | MIC (μg/ml) |
---|---|
Meropenem + this compound | 0.03 - 0.06 |
Imipenem + this compound | 0.25 |
Cefepime + this compound | 0.125 - 0.25 |
Aztreonam + this compound | 0.125 - 0.25 |
Ceftazidime + this compound | 0.125 - 0.25 |
Case Studies
-
TANGO II Trial :
- This Phase 3 trial compared meropenem-vaborbactam with best available therapy (BAT) for treating CRE infections.
- Results indicated a clinical cure rate of 65.6% for meropenem-vaborbactam compared to 33.3% for BAT at the end of treatment .
- The trial also reported a significant reduction in mortality rates associated with meropenem-vaborbactam treatment .
- Real-world Outcomes :
- Early Experience Analysis :
Q & A
Basic Research Questions
Q. What is the mechanism by which vaborbactam inhibits β-lactamases, and how does this inform experimental design for in vitro susceptibility testing?
this compound, a cyclic boronic acid pharmacophore, reversibly inhibits class A (e.g., KPC) and class C (AmpC) β-lactamases by forming covalent bonds with the catalytic serine residue . To assess its efficacy, in vitro studies measure reductions in carbapenem MIC values against β-lactamase-producing isolates. For example, this compound reduces meropenem MICs by ≥8-fold in KPC-producing Enterobacteriaceae . Standard methodologies include broth microdilution assays using CLSI guidelines, with this compound fixed at 8 µg/mL and meropenem serially diluted. Researchers must validate results across diverse enzyme subtypes (e.g., OXA-48, NDM) due to variable activity against class B/D enzymes .
Q. How are pharmacokinetic-pharmacodynamic (PK-PD) targets for this compound derived, and what models are used to validate them?
PK-PD targets for this compound are determined using non-clinical models. For instance, a 24-hour free-drug AUC:MIC ratio (fAUC:MIC) of 38 was identified as the target for 1-log10 bacterial reduction in murine thigh infection models . Hollow-fiber in vitro models further validate resistance suppression at fAUC:MIC ≥24 . Population pharmacokinetic models and Monte Carlo simulations (≥10,000 subjects) are employed to predict target attainment in humans, incorporating renal function variability . These methods confirm that a 3-hour infusion of meropenem/vaborbactam (2 g/2 g every 8 h) achieves target exposures in >90% of patients with normal renal function .
Advanced Research Questions
Q. How do clinical trial designs for meropenem-vaborbactam address heterogeneity in carbapenem-resistant Enterobacteriaceae (CRE) infections, and what statistical methods reconcile subgroup disparities?
Phase III trials like TANGO II used stratified randomization and modified intent-to-treat (MITT) analyses to evaluate CRE-specific outcomes. Sensitivity analyses were critical in addressing imbalances, such as prior antibiotic failure rates. For example, in patients without prior failure, meropenem-vaborbactam showed a 42.9% higher clinical cure rate (69.6% vs. 26.7%) and reduced mortality (4.3% vs. 33.3%) compared to best available therapy (BAT) . Covariate-adjusted logistic regression and propensity scoring are recommended to control for confounders like immunocompromised status .
Q. What methodologies resolve contradictions in this compound susceptibility data across β-lactamase subtypes?
Discrepancies in susceptibility (e.g., 99.1% for KPC vs. 1.7% for NDM-1 producers) require combinatorial testing and genomic validation. Researchers should:
- Perform whole-genome sequencing to confirm carbapenemase genes.
- Use checkerboard assays to assess synergy with meropenem against multidrug-resistant isolates.
- Apply epidemiological cutoffs (ECOFFs) to distinguish resistance mechanisms from intrinsic MIC variations . For OXA-48-like enzymes, susceptibility testing must account for regional enzyme variants, as 40.5% susceptibility may reflect strain-specific differences .
Q. How can population pharmacokinetic models optimize this compound dosing in renal impairment without compromising efficacy?
Population PK models derived from cUTI patients incorporate estimated glomerular filtration rate (eGFR) to adjust dosing. For eGFR 15–29 mL/min/1.73 m², simulations support dose reduction to 1 g/1 g every 8 h, maintaining fAUC:MIC >38 . Validation requires therapeutic drug monitoring (TDM) paired with LC-MS/MS quantification (linear range: 0.05–100 µg/mL) . Bayesian forecasting tools further individualize dosing in critically ill patients with fluctuating renal function .
Q. Methodological Considerations for Experimental Design
Q. What in vitro and in vivo models best predict this compound's resistance-suppression potential?
- In vitro: Hollow-fiber infection models (HFIM) simulate human PK profiles and test resistance emergence over 7–10 days. For meropenem-vaborbactam, HFIM shows resistance suppression at fAUC:MIC ≥24 .
- In vivo: Neutropenic murine thigh models quantify bactericidal activity (1-log10 kill) and correlate fAUC:MIC ratios with outcomes. Cross-validation with clinical isolates ensures translational relevance .
Q. How should researchers analyze PK-PD data when combining this compound with meropenem?
Use non-linear mixed-effects modeling (NONMEM) to account for drug-drug interactions. Key parameters include:
- Meropenem’s %fT>MIC (target ≥40% for bactericidal activity) .
- This compound’s fAUC:MIC ratio (target ≥38) . Joint PK-PD analyses must adjust for covariance between drugs, as this compound lacks intrinsic antibacterial activity but enhances meropenem stability .
Q. Tables for Key Data Reference
Parameter | This compound (2 g q8h) | Meropenem (2 g q8h) | Source |
---|---|---|---|
Plasma Protein Binding | 33% | 2% | |
Renal Clearance (L/h) | 8.9 | 7.8 | |
fAUC:MIC Target (Murine) | ≥38 | ≥40% fT>MIC | |
Susceptibility (KPC) | 99.1% | 9.3% (meropenem alone) |
Comparison with Similar Compounds
Comparison with Similar β-Lactamase Inhibitors
Structural and Mechanistic Differences
Compound | Class | Key Structural Features | Mechanism of Inhibition | Spectrum of Activity (β-Lactamases) |
---|---|---|---|---|
Vaborbactam | Cyclic boronic acid | Boronate ester ring, thiophene substituent | Reversible covalent binding to serine | Class A (KPC, SHV), Class C (AmpC) |
Avibactam | Diazabicyclooctane | DBO core with carboxy group | Covalent carbamylation of serine | Class A (KPC, ESBLs), Class C, some Class D |
Relebactam | Diazabicyclooctane | DBO core with methyl group | Covalent carbamylation of serine | Class A (KPC), Class C |
Clavulanic acid | β-Lactam | Clavam nucleus | Irreversible acylation of serine | Class A (TEM, SHV, ESBLs) |
This compound’s cyclic boronic acid structure enables unique interactions with β-lactamase active sites, such as hydrogen bonding with conserved residues (e.g., S130, T235, T237 in KPC-2) and hydrophobic contacts with the thiophene group . In contrast, avibactam’s DBO core relies on carbamylation and slower dissociation kinetics .
Spectrum of Activity and Resistance
Against Enterobacteriaceae:
- This compound + Meropenem: Reduces meropenem MICs by 8- to >1024-fold against KPC-producing strains. However, MICs increase when isolates harbor ompK35/ompK36 porin mutations or overexpress KPC enzymes (e.g., MIC ≥4/8 μg/mL for strains with dual porin loss + high blaKPC copy numbers) .
- Avibactam + Ceftazidime: Active against KPC and OXA-48-like enzymes but ineffective against metallo-β-lactamases (MBLs) and some ompK36-mutant K. pneumoniae. Cross-resistance between ceftazidime-avibactam and meropenem-vaborbactam is rare .
- Relebactam + Imipenem: Similar to this compound against KPC producers but less active against AmpC-overexpressing Enterobacterales .
Against Nonfermenters (Pseudomonas aeruginosa, Acinetobacter spp.):
- aeruginosa or Acinetobacter spp. due to intrinsic resistance mechanisms (e.g., efflux pumps, OprD porin loss, MBL production). MIC50/MIC90 values for meropenem-vaborbactam (0.5/8 μg/mL) mirror meropenem alone .
- In contrast, avibactam partially restores ceftazidime activity against AmpC-hyperproducing P. aeruginosa, but this compound shows negligible inhibition of PDC-type enzymes (IC50 >50 μM) .
Anaerobic and Gram-Positive Activity:
Pharmacokinetics/Pharmacodynamics (PK/PD)
Parameter | This compound | Meropenem | Avibactam |
---|---|---|---|
Protein Binding | 33% | 2% | 5–10% |
Half-Life | 1.3–1.7 h | 1.0 h | 2.2–2.7 h |
Renal Excretion | >90% unchanged | 70% unchanged | 80–90% unchanged |
AUC0–24 (free) | 290–410 μg·h/mL | 547 μg·h/mL | 340–450 μg·h/mL |
This compound and meropenem exhibit parallel PK profiles, enabling co-administration as a fixed-dose combination (2 g/2 g every 8 h). Dose adjustments are required for renal impairment (eGFR <50 mL/min/1.73 m²) .
Clinical Efficacy
- TANGO I Trial: Meropenem-vaborbactam demonstrated 98.4% clinical cure in complicated urinary tract infections (cUTIs), outperforming piperacillin-tazobactam (94.3%) .
- TANGO II Trial: In CRE infections, meropenem-vaborbactam achieved 65.6% clinical cure vs. 33.3% with best available therapy (e.g., polymyxins) .
Limitations and Resistance Concerns
- This compound lacks activity against MBLs (e.g., NDM, VIM) and Class D enzymes (OXA-48-like).
- Emergence of resistance due to porin mutations + KPC overexpression has been documented in vitro but remains rare clinically .
Preparation Methods
Enantioselective Synthesis of Key Intermediates
Preparation of S-3-Hydroxy-6-Oxohexanoic Acid Ester
The synthesis begins with the resolution of racemic β-hydroxy esters via lipase-mediated kinetic resolution, yielding enantiopure S-3-hydroxy-6-oxohexanoic acid tert-butyl ester (>99% ee) . This step leverages Candida antarctica lipase B to selectively acetylate the R-enantiomer, leaving the desired S-isomer unreacted. The process achieves 45–50% theoretical yield after hydrolysis, with optical purity confirmed by chiral HPLC .
Silyl Ether Protection
Subsequent protection of the hydroxyl group employs tert-butyldimethylsilyl chloride (TBSCl) in dichloromethane with imidazole as a base, yielding the silyl-protected intermediate (11) in 94% yield (Scheme 1) . Critical parameters include:
Parameter | Value |
---|---|
Temperature | 0–25°C |
Reaction Time | 1–2 h |
TBSCl Equivalents | 1.2 eq |
Solvent | CH₂Cl₂ |
¹H NMR (DMSO-d₆) analysis confirms complete protection, with characteristic tert-butyl singlet at δ 1.39 ppm and TBS methyl resonances at δ 0.04–0.02 ppm .
Hydroboration and Boronate Ester Formation
Iridium-Catalyzed Hydroboration
Regioselective hydroboration of the pentenoate side chain utilizes [Ir(COD)Cl]₂ catalysis (0.5 mol%) with pinacolborane, achieving >95% conversion to pinacol boronate (12) . The iridium catalyst directs anti-Markovnikov addition, with stereochemical control governed by the substrate’s existing chiral center.
Conversion to Pinanediol Boronate
Pinacol boronate (12) undergoes transesterification with (-)-pinanediol in toluene at reflux, yielding the configurationally stable pinanediol boronate (13) . This step enhances diastereomeric ratio (dr) from 85:15 to >99:1 through crystallographic resolution .
Matteson Homologation: Diastereoselective Chain Extension
The pivotal step in this compound synthesis involves dichloromethyllithium addition to boronate (13) under Matteson conditions:
Reaction Conditions
This generates the α-chloroboronic ester (14) with 85:15 dr in batch mode, improved to 98:2 dr via continuous flow processing . The flow system’s rapid heat dissipation prevents carbene formation from dichloromethyllithium decomposition, a major batch limitation.
Comparative Performance: Batch vs. Flow
Parameter | Batch Process | Flow Process |
---|---|---|
Temperature | -95°C | -78°C |
Reaction Time | 1 h | 20 s |
Yield | 72% | 97% |
Diastereomeric Ratio | 85:15 | 98:2 |
Final Functionalization and Deprotection
Displacement and Acylation
The chloro group in (14) undergoes stereospecific displacement with hexamethyldisilazide, followed by acylation with thiophene-2-acetyl chloride. This sequence installs the critical thiophene side chain while preserving boronate stereochemistry .
Global Deprotection
Simultaneous removal of silyl and tert-butyl protecting groups employs HCl in methanol/water (4:1), yielding this compound free acid. Final purification via reverse-phase HPLC achieves >99.5% purity, with residual solvents below ICH Q3C limits .
Continuous Flow Process Innovation
The Patheon/Thermo Fisher team revolutionized scale-up by implementing a continuous loop reactor for the Matteson step :
Flow System Design
-
Reactor Volume: 18 L (total)
-
Flow Rate: 12 L/min
-
Productivity: 2.4 kg/h
-
Cumulative Output: 580 kg/run
This configuration reduced zinc chloride fouling through dynamic slurry management, enabling cGMP production of multi-ton quantities for clinical trials .
Analytical Characterization and Quality Control
Critical quality attributes monitored during synthesis include:
Parameter | Specification | Method |
---|---|---|
Enantiomeric Excess | ≥99.5% ee | Chiral HPLC |
Boronate Content | 98–102% | ICP-MS |
Related Substances | ≤0.5% each | UHPLC-UV/ELSD |
CLSI-approved broth microdilution QC ranges ensure consistent potency against KPC-producing strains during API batch release .
Properties
IUPAC Name |
2-[(3R,6S)-2-hydroxy-3-[(2-thiophen-2-ylacetyl)amino]oxaborinan-6-yl]acetic acid | |
---|---|---|
Source | PubChem | |
URL | https://pubchem.ncbi.nlm.nih.gov | |
Description | Data deposited in or computed by PubChem | |
InChI |
InChI=1S/C12H16BNO5S/c15-11(7-9-2-1-5-20-9)14-10-4-3-8(6-12(16)17)19-13(10)18/h1-2,5,8,10,18H,3-4,6-7H2,(H,14,15)(H,16,17)/t8-,10-/m0/s1 | |
Source | PubChem | |
URL | https://pubchem.ncbi.nlm.nih.gov | |
Description | Data deposited in or computed by PubChem | |
InChI Key |
IOOWNWLVCOUUEX-WPRPVWTQSA-N | |
Source | PubChem | |
URL | https://pubchem.ncbi.nlm.nih.gov | |
Description | Data deposited in or computed by PubChem | |
Canonical SMILES |
B1(C(CCC(O1)CC(=O)O)NC(=O)CC2=CC=CS2)O | |
Source | PubChem | |
URL | https://pubchem.ncbi.nlm.nih.gov | |
Description | Data deposited in or computed by PubChem | |
Isomeric SMILES |
B1([C@H](CC[C@H](O1)CC(=O)O)NC(=O)CC2=CC=CS2)O | |
Source | PubChem | |
URL | https://pubchem.ncbi.nlm.nih.gov | |
Description | Data deposited in or computed by PubChem | |
Molecular Formula |
C12H16BNO5S | |
Source | PubChem | |
URL | https://pubchem.ncbi.nlm.nih.gov | |
Description | Data deposited in or computed by PubChem | |
DSSTOX Substance ID |
DTXSID901027690 | |
Record name | Vaborbactam | |
Source | EPA DSSTox | |
URL | https://comptox.epa.gov/dashboard/DTXSID901027690 | |
Description | DSSTox provides a high quality public chemistry resource for supporting improved predictive toxicology. | |
Molecular Weight |
297.14 g/mol | |
Source | PubChem | |
URL | https://pubchem.ncbi.nlm.nih.gov | |
Description | Data deposited in or computed by PubChem | |
CAS No. |
1360457-46-0 | |
Record name | (3R,6S)-2-Hydroxy-3-[[2-(2-thienyl)acetyl]amino]-1,2-oxaborinane-6-acetic acid | |
Source | CAS Common Chemistry | |
URL | https://commonchemistry.cas.org/detail?cas_rn=1360457-46-0 | |
Description | CAS Common Chemistry is an open community resource for accessing chemical information. Nearly 500,000 chemical substances from CAS REGISTRY cover areas of community interest, including common and frequently regulated chemicals, and those relevant to high school and undergraduate chemistry classes. This chemical information, curated by our expert scientists, is provided in alignment with our mission as a division of the American Chemical Society. | |
Explanation | The data from CAS Common Chemistry is provided under a CC-BY-NC 4.0 license, unless otherwise stated. | |
Record name | Vaborbactam [USAN:INN] | |
Source | ChemIDplus | |
URL | https://pubchem.ncbi.nlm.nih.gov/substance/?source=chemidplus&sourceid=1360457460 | |
Description | ChemIDplus is a free, web search system that provides access to the structure and nomenclature authority files used for the identification of chemical substances cited in National Library of Medicine (NLM) databases, including the TOXNET system. | |
Record name | Vaborbactam | |
Source | DrugBank | |
URL | https://www.drugbank.ca/drugs/DB12107 | |
Description | The DrugBank database is a unique bioinformatics and cheminformatics resource that combines detailed drug (i.e. chemical, pharmacological and pharmaceutical) data with comprehensive drug target (i.e. sequence, structure, and pathway) information. | |
Explanation | Creative Common's Attribution-NonCommercial 4.0 International License (http://creativecommons.org/licenses/by-nc/4.0/legalcode) | |
Record name | Vaborbactam | |
Source | EPA DSSTox | |
URL | https://comptox.epa.gov/dashboard/DTXSID901027690 | |
Description | DSSTox provides a high quality public chemistry resource for supporting improved predictive toxicology. | |
Record name | (3R,6S)-[2-Hydroxy-3-(2-thiophen-2-yl-acetylamino)-[1,2]oxaborinan-6-yl]-acetic acid | |
Source | European Chemicals Agency (ECHA) | |
URL | https://echa.europa.eu/information-on-chemicals | |
Description | The European Chemicals Agency (ECHA) is an agency of the European Union which is the driving force among regulatory authorities in implementing the EU's groundbreaking chemicals legislation for the benefit of human health and the environment as well as for innovation and competitiveness. | |
Explanation | Use of the information, documents and data from the ECHA website is subject to the terms and conditions of this Legal Notice, and subject to other binding limitations provided for under applicable law, the information, documents and data made available on the ECHA website may be reproduced, distributed and/or used, totally or in part, for non-commercial purposes provided that ECHA is acknowledged as the source: "Source: European Chemicals Agency, http://echa.europa.eu/". Such acknowledgement must be included in each copy of the material. ECHA permits and encourages organisations and individuals to create links to the ECHA website under the following cumulative conditions: Links can only be made to webpages that provide a link to the Legal Notice page. | |
Record name | VABORBACTAM | |
Source | FDA Global Substance Registration System (GSRS) | |
URL | https://gsrs.ncats.nih.gov/ginas/app/beta/substances/1C75676F8V | |
Description | The FDA Global Substance Registration System (GSRS) enables the efficient and accurate exchange of information on what substances are in regulated products. Instead of relying on names, which vary across regulatory domains, countries, and regions, the GSRS knowledge base makes it possible for substances to be defined by standardized, scientific descriptions. | |
Explanation | Unless otherwise noted, the contents of the FDA website (www.fda.gov), both text and graphics, are not copyrighted. They are in the public domain and may be republished, reprinted and otherwise used freely by anyone without the need to obtain permission from FDA. Credit to the U.S. Food and Drug Administration as the source is appreciated but not required. | |
Retrosynthesis Analysis
AI-Powered Synthesis Planning: Our tool employs the Template_relevance Pistachio, Template_relevance Bkms_metabolic, Template_relevance Pistachio_ringbreaker, Template_relevance Reaxys, Template_relevance Reaxys_biocatalysis model, leveraging a vast database of chemical reactions to predict feasible synthetic routes.
One-Step Synthesis Focus: Specifically designed for one-step synthesis, it provides concise and direct routes for your target compounds, streamlining the synthesis process.
Accurate Predictions: Utilizing the extensive PISTACHIO, BKMS_METABOLIC, PISTACHIO_RINGBREAKER, REAXYS, REAXYS_BIOCATALYSIS database, our tool offers high-accuracy predictions, reflecting the latest in chemical research and data.
Strategy Settings
Precursor scoring | Relevance Heuristic |
---|---|
Min. plausibility | 0.01 |
Model | Template_relevance |
Template Set | Pistachio/Bkms_metabolic/Pistachio_ringbreaker/Reaxys/Reaxys_biocatalysis |
Top-N result to add to graph | 6 |
Feasible Synthetic Routes
Disclaimer and Information on In-Vitro Research Products
Please be aware that all articles and product information presented on BenchChem are intended solely for informational purposes. The products available for purchase on BenchChem are specifically designed for in-vitro studies, which are conducted outside of living organisms. In-vitro studies, derived from the Latin term "in glass," involve experiments performed in controlled laboratory settings using cells or tissues. It is important to note that these products are not categorized as medicines or drugs, and they have not received approval from the FDA for the prevention, treatment, or cure of any medical condition, ailment, or disease. We must emphasize that any form of bodily introduction of these products into humans or animals is strictly prohibited by law. It is essential to adhere to these guidelines to ensure compliance with legal and ethical standards in research and experimentation.