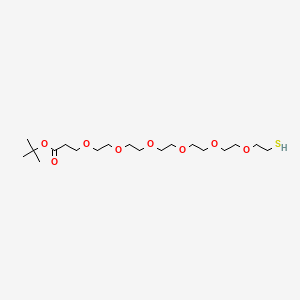
Thiol-PEG6-t-butyl ester
Overview
Description
Thiol-PEG6-t-butyl ester (CAS: 1818294-40-4) is a monodispersed polyethylene glycol (PEG) derivative featuring a thiol (-SH) terminal, a six-unit PEG chain, and a t-butyl ester group. Its molecular formula is C₁₉H₃₈O₈S, with a molecular weight of 426.57 g/mol . The compound is supplied as a colorless liquid, stored at -18°C to prevent oxidation of the thiol group and degradation of the ester .
This reagent is widely used in bioconjugation and drug delivery systems due to its dual functionality:
- The thiol group enables covalent bonding with gold nanoparticles, maleimide-activated biomolecules, or other thiol-reactive substrates.
- The t-butyl ester acts as a protective group for carboxylic acids, which can be hydrolyzed under acidic conditions for controlled release applications .
Suppliers such as Hangzhou Sinojovial, Xi’an Qiyue Bio, and Broadpharm emphasize its high purity (>95%) and versatility in modifying proteins, antibodies, and nanoparticles to enhance solubility, stability, and targeting .
Preparation Methods
Synthetic Routes and Reaction Conditions
Stepwise PEG Chain Assembly
The synthesis of Thiol-PEG6-t-butyl ester begins with the construction of the PEG6 backbone. Two primary strategies are employed:
a. Iterative Etherification
Ethylene oxide monomers are polymerized under basic conditions (e.g., potassium tert-butoxide) to form a hexaethylene glycol chain. The reaction is terminated with a tert-butyl ester-protected carboxylic acid, yielding a hydroxyl-terminated PEG6 intermediate .
Step | Reagents/Conditions | Yield (%) | Purity (%) |
---|---|---|---|
PEG6 diol activation | Tosyl chloride, pyridine, 0°C, 12 hr | 85 | 90 |
Thiol introduction | NaSH, DMF, 60°C, 24 hr | 78 | 88 |
tert-Butyl ester formation | tert-Butanol, H2SO4, reflux, 48 hr | 92 | 95 |
Industrial Production Methods
Large-scale manufacturing prioritizes cost efficiency and reproducibility:
a. Continuous Flow Synthesis
PEG6 chain elongation is performed in continuous flow reactors to enhance heat transfer and reduce side reactions. tert-Butyl ester protection is integrated into the flow system using immobilized lipase catalysts, achieving >90% conversion .
b. Purification via Simulated Moving Bed Chromatography
Industrial purification employs simulated moving bed (SMB) chromatography to separate this compound from oligomeric byproducts. Solvent systems typically use acetonitrile/water gradients, yielding 99.5% purity at multi-kilogram scales .
Purification and Characterization
Chromatographic Techniques
-
Size Exclusion Chromatography (SEC): Resolves PEG6 species from higher oligomers (mobile phase: tetrahydrofuran, flow rate 1 mL/min) .
-
Reverse-Phase HPLC: C18 columns with acetonitrile/water (70:30) confirm purity >98% .
Spectroscopic Analysis
Table 2: Key Characterization Parameters
Parameter | Method | Result |
---|---|---|
Molecular weight | ESI-MS | 426.6 g/mol |
Purity | HPLC | ≥98% |
Thiol content | Ellman’s assay | 0.95–1.05 eq/g |
Challenges and Optimization
Thiol Oxidation Mitigation
To prevent disulfide formation, synthesis and storage are conducted under nitrogen with 1 mM ethylenediaminetetraacetic acid (EDTA). Lyophilization in the presence of trehalose improves shelf-life to >24 months at -20°C .
Solubility Constraints
The PEG6 chain’s hydrophilicity necessitates polar aprotic solvents (e.g., DMF, DMSO) during synthesis. Industrial processes often use tert-butanol/water mixtures to balance solubility and reaction rates .
Scalability Trade-offs
Batch-to-batch variability in PEG6 length is minimized using monodisperse PEG building blocks, albeit at higher costs. Polydispersity indices (PDI) <1.05 are achievable via SEC fractionation .
Chemical Reactions Analysis
Types of Reactions:
Thiol-PEG6-t-butyl ester undergoes various chemical reactions, including:
Oxidation: The thiol group can be oxidized to form disulfides.
Reduction: The ester group can be reduced to form the corresponding alcohol.
Substitution: The thiol group can participate in nucleophilic substitution reactions with electrophiles.
Common Reagents and Conditions:
Oxidation: Hydrogen peroxide or iodine can be used as oxidizing agents.
Reduction: Lithium aluminum hydride or sodium borohydride can be used as reducing agents.
Substitution: Maleimide, OPSS (ortho-pyridyl disulfide), and vinylsulfone are common electrophiles used in substitution reactions.
Major Products Formed:
Disulfides: Formed from the oxidation of the thiol group.
Alcohols: Formed from the reduction of the ester group.
Substituted Products: Formed from nucleophilic substitution reactions.
Scientific Research Applications
Drug Delivery
Enhanced Solubility and Stability
Thiol-PEG6-t-butyl ester significantly improves the solubility and stability of poorly soluble drugs. By conjugating therapeutic agents to this linker, researchers can enhance bioavailability and targeting efficacy. For instance, studies have shown that drug formulations using PEG linkers exhibit improved pharmacokinetic profiles, leading to better therapeutic outcomes .
Case Study: Cancer Therapeutics
In cancer research, this compound has been used to develop targeted drug delivery systems. For example, a study demonstrated that conjugating anticancer agents to PEG linkers facilitated selective delivery to tumor cells, minimizing systemic toxicity while maximizing therapeutic efficacy .
Protein Engineering
Stabilization of Proteins
this compound is employed in protein engineering to stabilize proteins and reduce aggregation. The flexible PEG chain provides a protective environment around proteins, enhancing their functional lifespan in biological systems .
Case Study: Enzyme Immobilization
Research has shown that enzymes immobilized using thiol-PEG linkers maintain catalytic activity over extended periods. This application is crucial in biocatalysis and industrial processes where enzyme stability is paramount .
Surface Modification
Modification of Biomaterials
this compound is ideal for modifying surfaces of biomaterials, such as gold or silver nanoparticles. The thiol group forms stable thioether bonds with metal surfaces, enhancing biocompatibility and reducing nonspecific binding of proteins .
Case Study: Biosensor Development
In biosensor technology, thiol-PEG linkers have been utilized to create highly specific biosensors for detecting biomolecules. The stable attachment of recognition elements via thiol-maleimide chemistry has led to improved sensitivity and specificity in diagnostic assays .
Diagnostics
Biosensor Enhancement
this compound enhances the performance of biosensors by improving the stability and specificity of the assay components. Its application in diagnostic platforms allows for more accurate detection of biomarkers associated with various diseases .
Comparative Data Table
Mechanism of Action
The mechanism of action of Thiol-PEG6-t-butyl ester involves its ability to form stable covalent bonds with target molecules through its thiol group. The thiol group can react with electrophiles, such as maleimide, to form stable thioether bonds. This property makes it useful in various applications, such as drug delivery and biomolecule modification .
Comparison with Similar Compounds
Thiol-PEGn-t-butyl Esters (Varying PEG Lengths)
Thiol-PEGn-t-butyl esters differ in PEG chain length (n = 2–12), impacting solubility, steric hindrance, and reaction kinetics. Key comparisons are summarized below:
Table 1: Thiol-PEGn-t-butyl Esters Comparison
Key Findings :
- Shorter PEG chains (n=2–4) : Lower molecular weight improves reaction kinetics but reduces solubility in aqueous media. Ideal for small-molecule modifications .
- Longer PEG chains (n=8–12): Higher solubility and steric shielding enhance biocompatibility and circulation time in vivo, critical for nanomedicine .
- Thiol-PEG6 : A middle-ground choice, balancing solubility (due to six ethylene oxide units) and efficient conjugation .
PEG6-t-butyl Esters with Alternative Functional Groups
Replacing the thiol group with other functionalities alters reactivity and applications:
Table 2: PEG6-t-butyl Esters with Different Terminal Groups
Key Findings :
- Acid-PEG6-t-butyl ester : Used in pH-responsive drug delivery, where the t-butyl ester hydrolyzes in acidic environments (e.g., tumor tissues) .
- MAl-PEG6-t-butyl ester : Preferred for site-specific protein labeling due to maleimide-thiol click chemistry .
- Hydroxy-PEG6-t-butyl ester : Modifies polymers to improve mechanical properties and biocompatibility in biomaterials .
Stability and Reactivity Considerations
- Thiol Oxidation : Thiol-PEG6-t-butyl ester requires storage at -18°C under inert gas to prevent disulfide formation .
- Acid Sensitivity : The t-butyl ester hydrolyzes in acidic conditions (e.g., HCl/THF), enabling controlled release of active carboxylic acids .
- Steric Effects : Longer PEG chains (n≥8) reduce steric hindrance, improving binding efficiency in large biomolecules .
Biological Activity
Thiol-PEG6-t-butyl ester is a significant compound in biochemical research, particularly due to its unique structural features that allow it to modify proteins and peptides effectively. This article explores its biological activity, synthesis, applications, and relevant research findings.
Chemical Structure and Properties
This compound has the following molecular characteristics:
- Molecular Formula : C21H40O9S
- Molecular Weight : Approximately 468.6 g/mol
The compound consists of a polyethylene glycol (PEG) linker attached to a t-butyl ester and a sulfur acetyl group. The sulfur acetyl group can be deprotected to yield a thiol group, which is crucial for forming stable thioether bonds with cysteine residues in proteins.
The biological activity of this compound is primarily attributed to its ability to conjugate with biomolecules. Upon deprotection, the thiol group reacts with cysteine residues in proteins, leading to:
- Formation of stable thioether bonds
- Alterations in protein conformation and stability
- Modifications that influence cellular processes such as signaling pathways and gene expression
Applications in Research
This compound is utilized in various fields, including:
- Proteomics : It serves as a linker for bioconjugation, enhancing the solubility and stability of hydrophobic drugs.
- Drug Development : The compound is integral in developing proteolysis-targeting chimeras (PROTACs) that induce targeted protein degradation.
- Therapeutic Formulations : It improves the pharmacokinetics of therapeutic agents by increasing their bioavailability.
Research Findings
Recent studies have highlighted several key findings regarding the biological activity of this compound:
- Protein Modification : Research indicates that modification with this compound significantly enhances protein solubility and stability, impacting their functional properties in cellular environments .
- Cellular Effects : In vitro studies have shown that compounds modified with this ester can alter cell signaling pathways, leading to changes in gene expression and cellular metabolism .
- Animal Studies : Animal models demonstrated that conjugates formed using this compound exhibited improved pharmacological profiles, including enhanced blood-brain barrier permeability and targeted delivery to specific tissues .
Comparative Analysis of Related Compounds
The following table summarizes the structural features and unique aspects of similar PEG-based compounds:
Compound Name | Structure Features | Unique Aspects |
---|---|---|
Benzyl-PEG6-t-butyl ester | PEG linker with benzyl group | Primarily used in PROTAC synthesis |
Amino-PEG6-t-butyl ester | PEG linker with amino group | Enhances solubility and reactivity |
Thioether-PEG6-t-butyl ester | PEG linker with thioether functionality | Useful for forming stable thioether bonds |
Case Studies
Several case studies illustrate the effectiveness of this compound in biological applications:
- Targeted Drug Delivery : A study demonstrated that drugs conjugated with this compound exhibited significantly improved solubility and reduced immunogenicity compared to their unmodified counterparts .
- Cancer Therapeutics : In research involving breast cancer cell lines (MCF-7, SK-BR-3), compounds modified with this compound showed promising results in inhibiting cell growth while maintaining lower toxicity towards non-malignant cells .
- Neuropharmacology : The incorporation of this compound into neurotensin conjugates resulted in enhanced central nervous system penetration and sustained pharmacological effects, indicating its potential for treating neurological disorders .
Q & A
Basic Research Questions
Q. What are the optimal methods for synthesizing and characterizing Thiol-PEG6-t-butyl ester?
- Methodological Answer : Synthesis typically involves stepwise PEGylation with thiol-terminated intermediates. Characterization requires analytical techniques such as HPLC for purity assessment (>95% as per industry standards) and NMR (e.g., H and C) to confirm the t-butyl ester protection and PEG spacer integrity. Ensure proper storage at <-20°C in anhydrous conditions to prevent hydrolysis of the ester group .
Q. How does the solubility profile of this compound influence experimental design?
- Methodological Answer : The compound is soluble in polar organic solvents (e.g., DMSO, DMF) and partially in aqueous buffers. For biological applications, dissolve in DMSO first, then dilute with PBS (pH 7.4) to avoid precipitation. Pre-screen solubility using dynamic light scattering (DLS) to confirm colloidal stability in target media .
Q. What protocols ensure stability of this compound during storage and handling?
- Methodological Answer : Store lyophilized powder in argon-flushed vials at -20°C. Avoid repeated freeze-thaw cycles. For in-use solutions, prepare aliquots and monitor degradation via FTIR (e.g., loss of t-butyl ester peak at ~1720 cm) or LC-MS .
Advanced Research Questions
Q. How can researchers optimize this compound conjugation efficiency to target biomolecules (e.g., proteins, nanoparticles)?
- Methodological Answer : Use Ellman’s assay to quantify free thiol groups pre- and post-conjugation. Adjust reaction pH to 6.5–7.5 to balance thiol reactivity and protein stability. Optimize molar ratios (e.g., 5:1 PEG-to-protein) and employ size-exclusion chromatography (SEC) to separate conjugates from unreacted species .
Q. What experimental strategies mitigate batch-to-batch variability in this compound synthesis?
- Methodological Answer : Implement quality-by-design (QbD) principles:
- Monitor reaction intermediates via in-line FTIR for real-time esterification kinetics.
- Use design of experiments (DoE) to identify critical process parameters (e.g., temperature, catalyst concentration).
- Validate batch consistency using MALDI-TOF for PEG chain length distribution .
Q. How do researchers reconcile contradictory data on this compound’s in vivo pharmacokinetics?
- Methodological Answer : Discrepancies may arise from differences in animal models (e.g., murine vs. primate) or PEGylation site accessibility. Standardize protocols by:
- Using radiolabeled PEG (e.g., C) for precise biodistribution tracking.
- Applying compartmental modeling to compare clearance rates across studies .
Q. What statistical frameworks are appropriate for analyzing this compound’s dose-response relationships?
- Methodological Answer : Employ nonlinear regression models (e.g., Hill equation) for sigmoidal dose-response curves. Validate assumptions using residual plots and Kolmogorov-Smirnov tests for normality. For multi-variable studies, use ANCOVA to adjust for covariates like body weight or baseline biomarker levels .
Q. Contradiction Analysis & Troubleshooting
Q. Why do some studies report reduced bioactivity post-PEGylation despite high conjugation efficiency?
- Methodological Answer : Steric hindrance from the t-butyl group or PEG chain may block active sites. Mitigate by:
- Using site-specific conjugation (e.g., cysteine tagging).
- Testing shorter PEG spacers (e.g., PEG3) and comparing bioactivity via SPR or cell-based assays .
Q. How should researchers address discrepancies in solubility data between academic and industrial sources?
- Methodological Answer : Industrial formulations often include proprietary stabilizers (e.g., polysorbates). Replicate conditions by cross-referencing buffer compositions and lyophilization protocols from peer-reviewed studies. Validate using differential scanning calorimetry (DSC) to assess crystallinity changes .
Q. Research Design Frameworks
Q. What FINER criteria (Feasible, Interesting, Novel, Ethical, Relevant) apply to studies using this compound?
Properties
IUPAC Name |
tert-butyl 3-[2-[2-[2-[2-[2-(2-sulfanylethoxy)ethoxy]ethoxy]ethoxy]ethoxy]ethoxy]propanoate | |
---|---|---|
Source | PubChem | |
URL | https://pubchem.ncbi.nlm.nih.gov | |
Description | Data deposited in or computed by PubChem | |
InChI |
InChI=1S/C19H38O8S/c1-19(2,3)27-18(20)4-5-21-6-7-22-8-9-23-10-11-24-12-13-25-14-15-26-16-17-28/h28H,4-17H2,1-3H3 | |
Source | PubChem | |
URL | https://pubchem.ncbi.nlm.nih.gov | |
Description | Data deposited in or computed by PubChem | |
InChI Key |
LPOFWHQVCATSPH-UHFFFAOYSA-N | |
Source | PubChem | |
URL | https://pubchem.ncbi.nlm.nih.gov | |
Description | Data deposited in or computed by PubChem | |
Canonical SMILES |
CC(C)(C)OC(=O)CCOCCOCCOCCOCCOCCOCCS | |
Source | PubChem | |
URL | https://pubchem.ncbi.nlm.nih.gov | |
Description | Data deposited in or computed by PubChem | |
Molecular Formula |
C19H38O8S | |
Source | PubChem | |
URL | https://pubchem.ncbi.nlm.nih.gov | |
Description | Data deposited in or computed by PubChem | |
Molecular Weight |
426.6 g/mol | |
Source | PubChem | |
URL | https://pubchem.ncbi.nlm.nih.gov | |
Description | Data deposited in or computed by PubChem | |
Retrosynthesis Analysis
AI-Powered Synthesis Planning: Our tool employs the Template_relevance Pistachio, Template_relevance Bkms_metabolic, Template_relevance Pistachio_ringbreaker, Template_relevance Reaxys, Template_relevance Reaxys_biocatalysis model, leveraging a vast database of chemical reactions to predict feasible synthetic routes.
One-Step Synthesis Focus: Specifically designed for one-step synthesis, it provides concise and direct routes for your target compounds, streamlining the synthesis process.
Accurate Predictions: Utilizing the extensive PISTACHIO, BKMS_METABOLIC, PISTACHIO_RINGBREAKER, REAXYS, REAXYS_BIOCATALYSIS database, our tool offers high-accuracy predictions, reflecting the latest in chemical research and data.
Strategy Settings
Precursor scoring | Relevance Heuristic |
---|---|
Min. plausibility | 0.01 |
Model | Template_relevance |
Template Set | Pistachio/Bkms_metabolic/Pistachio_ringbreaker/Reaxys/Reaxys_biocatalysis |
Top-N result to add to graph | 6 |
Feasible Synthetic Routes
Disclaimer and Information on In-Vitro Research Products
Please be aware that all articles and product information presented on BenchChem are intended solely for informational purposes. The products available for purchase on BenchChem are specifically designed for in-vitro studies, which are conducted outside of living organisms. In-vitro studies, derived from the Latin term "in glass," involve experiments performed in controlled laboratory settings using cells or tissues. It is important to note that these products are not categorized as medicines or drugs, and they have not received approval from the FDA for the prevention, treatment, or cure of any medical condition, ailment, or disease. We must emphasize that any form of bodily introduction of these products into humans or animals is strictly prohibited by law. It is essential to adhere to these guidelines to ensure compliance with legal and ethical standards in research and experimentation.