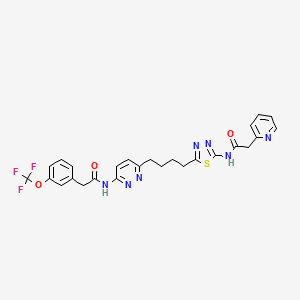
CB-839
Overview
Description
Telaglenastat is a potent, selective, and orally bioavailable inhibitor of glutaminase, an enzyme that plays a critical role in the metabolism of glutamine. This compound has shown promise in the treatment of various cancers by targeting the metabolic pathways that cancer cells rely on for growth and survival .
Mechanism of Action
Target of Action
Telaglenastat, also known as CB-839, is a potent, selective, and orally bioavailable inhibitor of glutaminase (GLS) . GLS is a mitochondrial enzyme that plays a crucial role in the metabolism of glutamine, a non-essential amino acid that is vital for cellular growth and proliferation .
Mode of Action
Telaglenastat selectively and irreversibly inhibits GLS, which is essential for the conversion of the amino acid glutamine into glutamate . By blocking glutamine utilization, proliferation in rapidly growing cells is impaired .
Biochemical Pathways
The inhibition of GLS by Telaglenastat disrupts the metabolic pathways that cancer cells heavily rely on . This results in a decrease in glutamine consumption, which is concomitant with a decrease in the production of glutamate and other glutamine-derived metabolites . This disruption is consistent with GLS inhibition .
Pharmacokinetics
Telaglenastat has demonstrated favorable pharmacokinetics (PK) and pharmacodynamics (PDs) in early clinical studies . It is orally bioavailable , and its IC50 value for recombinant human GAC is 24 nM .
Result of Action
Telaglenastat has shown antitumor activity . In vitro studies have shown that Telaglenastat treatment improved the cytotoxic activity of autologous tumor-infiltrating lymphocytes (TILs) on patient-derived melanoma cells . In vivo, Telaglenastat treatment activated melanoma antigen–specific T cells and improved their tumor-killing activity . Additionally, the combination of Telaglenastat with anti-PD1 or anti-CTLA4 antibodies increased tumor infiltration by effector T cells and improved the antitumor activity of these checkpoint inhibitors .
Action Environment
The action of Telaglenastat can be influenced by the tumor microenvironment. For instance, in vitro TIL:tumor coculture studies have shown that Telaglenastat treatment decreased the conversion of glutamine to alpha-ketoglutarate (αKGA) more potently in tumor cells versus TILs . These results suggest that Telaglenastat may improve immune function in a tumor microenvironment by differentially altering tumor and immune cell metabolism .
Biochemical Analysis
Biochemical Properties
Telaglenastat plays a significant role in biochemical reactions by inhibiting glutaminase, a mitochondrial enzyme essential for the conversion of the amino acid glutamine into glutamate . This inhibition leads to decreased extracellular acidification rates and oxygen consumption rates , disrupting the metabolic pathways that cancer cells heavily rely on .
Cellular Effects
Telaglenastat has shown to have profound effects on various types of cells and cellular processes. It has been found to improve the cytotoxic activity of autologous tumor-infiltrating lymphocytes (TILs) on patient-derived melanoma cells . Moreover, it has demonstrated antiproliferative activity in a triple-negative breast cancer (TNBC) cell line, HCC-1806 .
Molecular Mechanism
The molecular mechanism of Telaglenastat involves the inhibition of glutaminase, thereby blocking the conversion of glutamine to glutamate . This disruption in glutamine metabolism results in decreased production of glutamate and other glutamine-derived metabolites, consistent with glutaminase inhibition .
Temporal Effects in Laboratory Settings
In laboratory settings, Telaglenastat has shown to decrease the conversion of glutamine to alpha-ketoglutarate (αKGA) more potently in tumor cells versus TILs in cocultures . This suggests that Telaglenastat may improve immune function in a tumor microenvironment by differentially altering tumor and immune cell metabolism over time .
Dosage Effects in Animal Models
In animal models, Telaglenastat has shown to suppress tumor growth by 61% relative to vehicle control when administered as a single agent at a dosage of 200 mg/kg . It has also been observed to activate melanoma antigen–specific T cells and improve their tumor-killing activity .
Metabolic Pathways
Telaglenastat is involved in the metabolic pathway of glutamine to glutamate conversion . By inhibiting glutaminase, it disrupts this pathway, leading to a decrease in glutamine consumption and the production of glutamate and other glutamine-derived metabolites .
Transport and Distribution
While specific transporters or binding proteins for Telaglenastat have not been explicitly mentioned in the literature, its oral bioavailability suggests that it can be effectively absorbed and distributed within the body .
Subcellular Localization
The exact subcellular localization of Telaglenastat is not explicitly stated in the literature. Given that it inhibits glutaminase, a mitochondrial enzyme , it can be inferred that Telaglenastat likely localizes to the mitochondria where it exerts its inhibitory effects.
Preparation Methods
The synthesis of Telaglenastat involves several steps, including the formation of key intermediates and the final coupling reactions. The synthetic routes typically involve the use of specific reagents and catalysts to achieve the desired chemical transformations. Industrial production methods focus on optimizing these synthetic routes to ensure high yield and purity of the final product .
Chemical Reactions Analysis
Telaglenastat undergoes various chemical reactions, including:
Oxidation: This reaction involves the addition of oxygen or the removal of hydrogen, often using oxidizing agents.
Reduction: This reaction involves the addition of hydrogen or the removal of oxygen, typically using reducing agents.
Substitution: This reaction involves the replacement of one functional group with another, often using nucleophiles or electrophiles.
Common reagents and conditions used in these reactions include oxidizing agents like hydrogen peroxide, reducing agents like sodium borohydride, and nucleophiles like ammonia. The major products formed from these reactions depend on the specific conditions and reagents used .
Scientific Research Applications
Telaglenastat has a wide range of scientific research applications, including:
Chemistry: Used as a tool to study glutaminase activity and its role in cellular metabolism.
Biology: Helps in understanding the metabolic pathways involved in cancer cell proliferation.
Medicine: Investigated for its potential to enhance the efficacy of immunotherapies and other cancer treatments.
Industry: Utilized in the development of new therapeutic agents targeting metabolic pathways
Comparison with Similar Compounds
Telaglenastat is unique in its high selectivity and potency as a glutaminase inhibitor. Similar compounds include:
6-diazo-5-oxonorleucine (DON): An older glutaminase inhibitor with broader activity.
bis-2-(5-phenylacetamido-1,3,4-thiadiazol-2-yl) ethyl sulphide (BPTES): Another selective glutaminase inhibitor with different kinetic properties.
5-(3-Bromo-4-(dimethylamino)phenyl)-2,2-dimethyl-2,3,5,6-tetrahydrobenzo[a]phenanthridin-4(1H)-one (968): A compound with similar inhibitory effects but different bioavailability.
Telaglenastat stands out due to its favorable pharmacokinetic and pharmacodynamic profile, making it a promising candidate for further clinical development .
Properties
IUPAC Name |
N-[6-[4-[5-[(2-pyridin-2-ylacetyl)amino]-1,3,4-thiadiazol-2-yl]butyl]pyridazin-3-yl]-2-[3-(trifluoromethoxy)phenyl]acetamide | |
---|---|---|
Source | PubChem | |
URL | https://pubchem.ncbi.nlm.nih.gov | |
Description | Data deposited in or computed by PubChem | |
InChI |
InChI=1S/C26H24F3N7O3S/c27-26(28,29)39-20-9-5-6-17(14-20)15-22(37)31-21-12-11-18(33-34-21)7-1-2-10-24-35-36-25(40-24)32-23(38)16-19-8-3-4-13-30-19/h3-6,8-9,11-14H,1-2,7,10,15-16H2,(H,31,34,37)(H,32,36,38) | |
Source | PubChem | |
URL | https://pubchem.ncbi.nlm.nih.gov | |
Description | Data deposited in or computed by PubChem | |
InChI Key |
PRAAPINBUWJLGA-UHFFFAOYSA-N | |
Source | PubChem | |
URL | https://pubchem.ncbi.nlm.nih.gov | |
Description | Data deposited in or computed by PubChem | |
Canonical SMILES |
C1=CC=NC(=C1)CC(=O)NC2=NN=C(S2)CCCCC3=NN=C(C=C3)NC(=O)CC4=CC(=CC=C4)OC(F)(F)F | |
Source | PubChem | |
URL | https://pubchem.ncbi.nlm.nih.gov | |
Description | Data deposited in or computed by PubChem | |
Molecular Formula |
C26H24F3N7O3S | |
Source | PubChem | |
URL | https://pubchem.ncbi.nlm.nih.gov | |
Description | Data deposited in or computed by PubChem | |
Molecular Weight |
571.6 g/mol | |
Source | PubChem | |
URL | https://pubchem.ncbi.nlm.nih.gov | |
Description | Data deposited in or computed by PubChem | |
CAS No. |
1439399-58-2 | |
Record name | Telaglenastat [USAN] | |
Source | ChemIDplus | |
URL | https://pubchem.ncbi.nlm.nih.gov/substance/?source=chemidplus&sourceid=1439399582 | |
Description | ChemIDplus is a free, web search system that provides access to the structure and nomenclature authority files used for the identification of chemical substances cited in National Library of Medicine (NLM) databases, including the TOXNET system. | |
Record name | Telaglenastat | |
Source | DrugBank | |
URL | https://www.drugbank.ca/drugs/DB15232 | |
Description | The DrugBank database is a unique bioinformatics and cheminformatics resource that combines detailed drug (i.e. chemical, pharmacological and pharmaceutical) data with comprehensive drug target (i.e. sequence, structure, and pathway) information. | |
Explanation | Creative Common's Attribution-NonCommercial 4.0 International License (http://creativecommons.org/licenses/by-nc/4.0/legalcode) | |
Record name | TELAGLENASTAT | |
Source | FDA Global Substance Registration System (GSRS) | |
URL | https://gsrs.ncats.nih.gov/ginas/app/beta/substances/U6CL98GLP4 | |
Description | The FDA Global Substance Registration System (GSRS) enables the efficient and accurate exchange of information on what substances are in regulated products. Instead of relying on names, which vary across regulatory domains, countries, and regions, the GSRS knowledge base makes it possible for substances to be defined by standardized, scientific descriptions. | |
Explanation | Unless otherwise noted, the contents of the FDA website (www.fda.gov), both text and graphics, are not copyrighted. They are in the public domain and may be republished, reprinted and otherwise used freely by anyone without the need to obtain permission from FDA. Credit to the U.S. Food and Drug Administration as the source is appreciated but not required. | |
Retrosynthesis Analysis
AI-Powered Synthesis Planning: Our tool employs the Template_relevance Pistachio, Template_relevance Bkms_metabolic, Template_relevance Pistachio_ringbreaker, Template_relevance Reaxys, Template_relevance Reaxys_biocatalysis model, leveraging a vast database of chemical reactions to predict feasible synthetic routes.
One-Step Synthesis Focus: Specifically designed for one-step synthesis, it provides concise and direct routes for your target compounds, streamlining the synthesis process.
Accurate Predictions: Utilizing the extensive PISTACHIO, BKMS_METABOLIC, PISTACHIO_RINGBREAKER, REAXYS, REAXYS_BIOCATALYSIS database, our tool offers high-accuracy predictions, reflecting the latest in chemical research and data.
Strategy Settings
Precursor scoring | Relevance Heuristic |
---|---|
Min. plausibility | 0.01 |
Model | Template_relevance |
Template Set | Pistachio/Bkms_metabolic/Pistachio_ringbreaker/Reaxys/Reaxys_biocatalysis |
Top-N result to add to graph | 6 |
Feasible Synthetic Routes
Q1: What is the primary mechanism of action of Telaglenastat?
A1: Telaglenastat exerts its anti-tumor effects by selectively inhibiting GLS1, the first enzyme in the glutaminolysis pathway. [, , ] This inhibition blocks the conversion of glutamine to glutamate, disrupting a critical metabolic pathway that tumor cells rely on for energy, biosynthesis, and redox balance. [, , , , , , ]
Q2: What are the downstream effects of GLS1 inhibition by Telaglenastat?
A2: Inhibition of GLS1 by Telaglenastat leads to a cascade of downstream effects in tumor cells, including:
- Depletion of Glutamate and α-Ketoglutarate: Reduces the availability of glutamate, a precursor for glutathione synthesis and a key substrate for the tricarboxylic acid (TCA) cycle. This disrupts redox balance and energy production in tumor cells. [, , , , , ]
- Impaired TCA Cycle Function: Depletion of glutamate and α-ketoglutarate impairs the TCA cycle, a central metabolic hub for energy production and biosynthesis. [, , ]
- Disruption of Nucleotide Synthesis: Limits the availability of glutamine-derived nitrogen for nucleotide biosynthesis, hindering DNA replication and repair processes crucial for tumor cell proliferation. [, ]
- Induction of Oxidative Stress: Inhibition of glutathione synthesis increases oxidative stress, further compromising tumor cell survival. [, , , ]
Q3: How does the effect of Telaglenastat on tumor cells differ from its effect on normal cells?
A3: While both tumor cells and normal cells utilize glutamine, tumor cells often exhibit heightened glutamine dependence, making them more susceptible to GLS1 inhibition by Telaglenastat. [, , , ] This differential sensitivity forms the basis for the therapeutic window of Telaglenastat.
Q4: What preclinical models have been used to evaluate the efficacy of Telaglenastat?
A4: Telaglenastat has shown promising anti-tumor activity in a variety of preclinical models, including:
- In vitro studies: Cell lines derived from various cancer types, including melanoma, renal cell carcinoma (RCC), multiple myeloma, and osteosarcoma. [, , , , , , , , , , ]
- In vivo studies: Xenograft mouse models implanted with human tumor cells, demonstrating tumor growth inhibition and improved survival. [, , , , , , , , , , , ]
Q5: What clinical trials have been conducted with Telaglenastat, and what are the key findings?
A5: Telaglenastat has been investigated in several clinical trials, both as monotherapy and in combination with other anti-cancer agents, for a range of hematological malignancies and solid tumors. Key findings from these trials include:
- Phase I Trials: Established the safety, tolerability, pharmacokinetic profile, and recommended phase II dose of Telaglenastat in patients with advanced solid tumors. [, ]
- Phase Ib/II Trials: Demonstrated promising anti-tumor activity and a manageable safety profile in combination with azacitidine in patients with advanced myelodysplastic syndrome (MDS). [, , ]
- Ongoing Trials: Telaglenastat is currently being evaluated in clinical trials for various cancer types, including lung cancer, multiple myeloma, and osteosarcoma, both as monotherapy and in combination with other therapies. [, , , , ]
Q6: What are the potential biomarkers for predicting response to Telaglenastat therapy?
A6: Research is ongoing to identify robust biomarkers for predicting response to Telaglenastat. Potential biomarkers under investigation include:
- GLS1 expression levels: Elevated GLS1 expression in tumors may correlate with increased sensitivity to Telaglenastat. [, , , , ]
- Glutamine transporter expression: High expression of glutamine transporters, such as SLC38A1, may indicate increased glutamine dependency and potential responsiveness to Telaglenastat. []
- Metabolic profiling: Identifying specific metabolic signatures associated with sensitivity or resistance to Telaglenastat could guide patient selection and treatment strategies. [, , , ]
Q7: What are the potential mechanisms of resistance to Telaglenastat?
A7: Understanding and overcoming resistance to Telaglenastat is crucial for maximizing its clinical benefit. Potential mechanisms of resistance include:
- Metabolic rewiring: Tumor cells may adapt to GLS1 inhibition by upregulating alternative metabolic pathways, such as increased glucose uptake and utilization, or by utilizing other amino acids as fuel sources. [, , , , ]
- GLS1 mutations: Mutations in the GLS1 gene could potentially reduce the binding affinity of Telaglenastat, leading to decreased drug efficacy. [, , , ]
- Tumor heterogeneity: The presence of diverse subpopulations within a tumor, some of which may be inherently resistant to Telaglenastat, could contribute to treatment failure. [, ]
Q8: What are the potential strategies to overcome resistance to Telaglenastat?
A8: Several strategies are being explored to overcome resistance to Telaglenastat:
- Combination therapies: Combining Telaglenastat with other anti-cancer agents, such as those targeting alternative metabolic pathways or those with synergistic mechanisms of action, may enhance efficacy and delay or prevent resistance. [, , , , , , , , , , , , , ]
- Development of next-generation GLS1 inhibitors: Designing inhibitors with improved potency, selectivity, and pharmacokinetic properties could potentially overcome some resistance mechanisms. [, , , ]
- Patient stratification based on biomarkers: Identifying biomarkers that predict response to Telaglenastat could enable the selection of patients most likely to benefit from therapy, potentially improving clinical outcomes. [, , , , , ]
Disclaimer and Information on In-Vitro Research Products
Please be aware that all articles and product information presented on BenchChem are intended solely for informational purposes. The products available for purchase on BenchChem are specifically designed for in-vitro studies, which are conducted outside of living organisms. In-vitro studies, derived from the Latin term "in glass," involve experiments performed in controlled laboratory settings using cells or tissues. It is important to note that these products are not categorized as medicines or drugs, and they have not received approval from the FDA for the prevention, treatment, or cure of any medical condition, ailment, or disease. We must emphasize that any form of bodily introduction of these products into humans or animals is strictly prohibited by law. It is essential to adhere to these guidelines to ensure compliance with legal and ethical standards in research and experimentation.