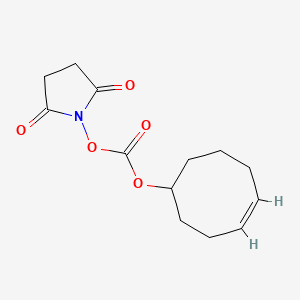
TCO-NHS ester
Overview
Description
TCO-NHS ester, also known as trans-cyclooctene-N-hydroxysuccinimide ester, is a compound widely used in the field of bioorthogonal chemistry. It is an alkyl/ether-based linker that plays a crucial role in the synthesis of proteolysis-targeting chimeras (PROTACs) and other bioconjugation applications. This compound is particularly valued for its ability to form stable covalent bonds with primary amines, making it an essential tool for protein labeling and modification .
Mechanism of Action
Target of Action
Trans-Cyclooctene-N-Hydroxysuccinimide Ester, commonly known as TCO-NHS ester, is a popular reagent used to label amine-containing compounds or biomolecules . The primary targets of this compound are primary amines, which are common functional groups present in virtually all proteins, peptides, and a host of other macromolecules . They exist at the N-terminus of each polypeptide chain (α-amino group) and in the side-chain of lysine amino acid residues (ε-amino group) .
Mode of Action
This compound reacts specifically and efficiently with a primary amine (e.g., side chain of lysine residues or aminosilane-coated surfaces) at pH 7-9 to form a covalent bond . This reaction is facilitated by the amine-reactive N-Hydroxysuccinimide (NHS) ester present in the this compound . The trans-cyclooctene (TCO) part of the this compound reacts rapidly with tetrazines through Diels–Alder reactions, a type of bioorthogonal, copper-free click chemistry .
Biochemical Pathways
The this compound, through its interaction with primary amines, plays a crucial role in bioconjugation and protein labeling . It enables fast click chemistry with tetrazine , facilitating the formation of stable amide bonds between two target molecules . This process is part of a broader biochemical pathway known as bioorthogonal cycloaddition reaction .
Pharmacokinetics
It’s worth noting that this compound shows favorable physiochemical properties for live-cell and in vivo labeling , suggesting that it may have good bioavailability.
Result of Action
The result of the action of this compound is the formation of a stable amide bond between the labeling reagent and the target molecule . This allows for the efficient and specific detection of targets like proteins or nucleic acids using affinity labeling reagents . In the context of super-resolution fluorescence imaging, this enables highly multiplexed imaging of target molecules .
Action Environment
The action of this compound is influenced by environmental factors such as pH. The reaction between this compound and primary amines occurs specifically and efficiently at pH 7-9 . Furthermore, the TCO functional group remains stable in aqueous buffered media for weeks at 4°C, pH 7.5 , indicating that the stability of this compound is influenced by temperature and pH. The storage condition for this compound is typically at -20°C .
Biochemical Analysis
Biochemical Properties
TCO-NHS ester plays a significant role in biochemical reactions due to its ability to react specifically and efficiently with a primary amine . This property allows it to form covalent bonds with biomolecules such as the side chain of lysine residues or aminosilane-coated surfaces .
Cellular Effects
The effects of this compound on cells and cellular processes are primarily related to its role in protein labeling. By forming covalent bonds with proteins, this compound can influence cell function, including impacts on cell signaling pathways, gene expression, and cellular metabolism .
Molecular Mechanism
At the molecular level, this compound exerts its effects through its ability to form covalent bonds with biomolecules. This includes binding interactions with proteins, enzyme inhibition or activation, and changes in gene expression .
Temporal Effects in Laboratory Settings
In laboratory settings, the effects of this compound can change over time. This includes information on the product’s stability, degradation, and any long-term effects on cellular function observed in in vitro or in vivo studies .
Dosage Effects in Animal Models
The effects of this compound can vary with different dosages in animal models. This could include any threshold effects observed in these studies, as well as any toxic or adverse effects at high doses .
Metabolic Pathways
This compound is involved in metabolic pathways that include interactions with enzymes or cofactors . This could also include any effects on metabolic flux or metabolite levels .
Transport and Distribution
This compound is transported and distributed within cells and tissues. This could include any transporters or binding proteins that it interacts with, as well as any effects on its localization or accumulation .
Subcellular Localization
The subcellular localization of this compound and any effects on its activity or function could include any targeting signals or post-translational modifications that direct it to specific compartments or organelles .
Preparation Methods
Synthetic Routes and Reaction Conditions
The synthesis of TCO-NHS ester typically involves the reaction of trans-cyclooctene with N-hydroxysuccinimide (NHS) in the presence of a coupling agent such as dicyclohexylcarbodiimide (DCC). The reaction is carried out under anhydrous conditions to prevent hydrolysis of the NHS ester. The general reaction scheme is as follows:
- Dissolve trans-cyclooctene and NHS in an anhydrous solvent such as dichloromethane.
- Add DCC to the reaction mixture to activate the carboxyl group of trans-cyclooctene.
- Stir the reaction mixture at room temperature for several hours.
- Filter the reaction mixture to remove the dicyclohexylurea byproduct.
- Purify the product using column chromatography to obtain this compound .
Industrial Production Methods
In industrial settings, the production of this compound follows similar synthetic routes but on a larger scale. The process involves the use of automated reactors and purification systems to ensure high yield and purity. The reaction conditions are optimized to minimize byproducts and maximize the efficiency of the synthesis .
Chemical Reactions Analysis
Types of Reactions
TCO-NHS ester primarily undergoes substitution reactions, where the NHS ester group reacts with primary amines to form stable amide bonds. This reaction is highly specific and efficient, making it ideal for bioconjugation applications .
Common Reagents and Conditions
Reagents: Primary amines (e.g., lysine residues on proteins), anhydrous solvents (e.g., dimethyl sulfoxide, dichloromethane), and coupling agents (e.g., DCC).
Conditions: The reaction is typically carried out at a pH range of 7-9 and at room temperature.
Major Products
The major product formed from the reaction of this compound with primary amines is a stable amide bond. This covalent bond is highly stable and resistant to hydrolysis, making it suitable for various bioconjugation applications .
Scientific Research Applications
TCO-NHS ester has a wide range of applications in scientific research, particularly in the fields of chemistry, biology, medicine, and industry. Some of its key applications include:
Protein Labeling: This compound is used to label proteins with fluorescent dyes or other tags for imaging and detection purposes.
PROTAC Synthesis: It serves as a linker in the synthesis of PROTACs, which are molecules designed to target and degrade specific proteins within cells.
Drug Delivery: This compound is used to conjugate drugs to targeting molecules, enhancing the specificity and efficacy of drug delivery systems.
Bioconjugation: It is employed in the conjugation of biomolecules such as antibodies, peptides, and nucleic acids for various research and therapeutic applications.
Comparison with Similar Compounds
TCO-NHS ester is unique in its ability to form stable covalent bonds with primary amines, making it highly valuable for bioconjugation applications. Similar compounds include:
TCO-PEG4-NHS ester: This compound has a polyethylene glycol (PEG) spacer, which provides increased water solubility and reduced aggregation compared to this compound.
TCO-PEG3-maleimide: This compound contains a maleimide group, allowing it to react with thiol groups in addition to primary amines.
TCO-PEG4-DBCO: This compound contains a dibenzocyclooctyne (DBCO) group, enabling it to participate in strain-promoted azide-alkyne cycloaddition reactions.
Each of these compounds has unique properties that make them suitable for specific applications, but this compound remains a versatile and widely used tool in bioorthogonal chemistry.
Biological Activity
Trans-Cyclooctene (TCO)-NHS ester is a versatile chemical compound widely used in bioconjugation and bioorthogonal chemistry. Its unique properties enable it to react specifically with primary amines, making it a valuable tool in various biological applications, including protein labeling and drug delivery systems. This article delves into the biological activity of TCO-NHS ester, supported by research findings, case studies, and data tables.
This compound is an amine-reactive building block characterized by its ability to form covalent bonds with primary amines at physiological pH (7-9). The reaction proceeds via the formation of a stable amide bond, which is crucial for the conjugation of biomolecules such as proteins and antibodies. The compound has a molecular weight of 267.28 g/mol and a chemical formula of C13H17NO5 .
Key Properties:
- Molecular Weight: 267.28 g/mol
- Chemical Formula: C13H17NO5
- Solubility: Soluble in DCM, chloroform, DMSO, and DMF
- Purity: Greater than 90% as determined by H NMR .
Applications in Biological Research
This compound is primarily utilized in:
- Protein Conjugation: It facilitates the conjugation of proteins to small molecules, antibodies, or oligonucleotides.
- Radiolabeling: It is employed in the preparation of radiolabeled compounds for imaging studies, particularly using fluorine-18.
- Surface Modification: this compound can modify surfaces for enhanced biocompatibility and functionality in biomaterials .
Case Study 1: Protein Labeling with this compound
In a study evaluating the efficacy of this compound for protein labeling, researchers demonstrated that varying concentrations of this compound impacted the degree of conjugation achieved. For instance, using 250 equivalents of TCO resulted in an average loading of 1.5 TCOs per nanobody (NB), while higher concentrations led to decreased solubility and aggregation . This study highlights the importance of optimizing reagent concentrations to achieve desired labeling efficiency without compromising protein integrity.
Case Study 2: Biodistribution Studies
Another significant application involved the use of this compound in labeling nanobodies for biodistribution studies in Long Evans rats. The labeled proteins were tracked using positron emission tomography (PET). Results indicated rapid excretion through the liver, with no significant brain uptake observed. This finding suggests that while TCO-NBS can effectively label proteins for tracking, their lipophilicity may influence biodistribution patterns .
Comparative Data Table: this compound vs. Other Conjugation Methods
Property | This compound | Conventional NHS Ester | Click Chemistry (Azide) |
---|---|---|---|
Reaction Type | Bioorthogonal | Non-bioorthogonal | Bioorthogonal |
Reaction Rate (k) | >800 M⁻¹s⁻¹ | Moderate | High |
Selectivity | High | Moderate | High |
Solubility | Good | Variable | Good |
Applications | Broad | Limited | Broad |
Research Findings on Biological Activity
Recent research has underscored the significance of this compound in enhancing the delivery and efficacy of therapeutic agents. The inverse-electron demand Diels-Alder reaction between TCO and tetrazines has shown exceptional kinetics and selectivity, enabling precise targeting in complex biological environments. This feature is particularly advantageous for applications involving low-abundance biomolecules .
Properties
IUPAC Name |
[(4E)-cyclooct-4-en-1-yl] (2,5-dioxopyrrolidin-1-yl) carbonate | |
---|---|---|
Source | PubChem | |
URL | https://pubchem.ncbi.nlm.nih.gov | |
Description | Data deposited in or computed by PubChem | |
InChI |
InChI=1S/C13H17NO5/c15-11-8-9-12(16)14(11)19-13(17)18-10-6-4-2-1-3-5-7-10/h1-2,10H,3-9H2/b2-1+ | |
Source | PubChem | |
URL | https://pubchem.ncbi.nlm.nih.gov | |
Description | Data deposited in or computed by PubChem | |
InChI Key |
OUGQJOKGFAIFAQ-OWOJBTEDSA-N | |
Source | PubChem | |
URL | https://pubchem.ncbi.nlm.nih.gov | |
Description | Data deposited in or computed by PubChem | |
Canonical SMILES |
C1CC=CCCC(C1)OC(=O)ON2C(=O)CCC2=O | |
Source | PubChem | |
URL | https://pubchem.ncbi.nlm.nih.gov | |
Description | Data deposited in or computed by PubChem | |
Isomeric SMILES |
C1C/C=C/CCC(C1)OC(=O)ON2C(=O)CCC2=O | |
Source | PubChem | |
URL | https://pubchem.ncbi.nlm.nih.gov | |
Description | Data deposited in or computed by PubChem | |
Molecular Formula |
C13H17NO5 | |
Source | PubChem | |
URL | https://pubchem.ncbi.nlm.nih.gov | |
Description | Data deposited in or computed by PubChem | |
Molecular Weight |
267.28 g/mol | |
Source | PubChem | |
URL | https://pubchem.ncbi.nlm.nih.gov | |
Description | Data deposited in or computed by PubChem | |
CAS No. |
1191901-33-3 | |
Record name | CYCLOOCT-4-ENYL 2,5-DIOXOPYRROLIDIN-1-YL CARBONATE, (E)- | |
Source | FDA Global Substance Registration System (GSRS) | |
URL | https://gsrs.ncats.nih.gov/ginas/app/beta/substances/2BX65M7XV6 | |
Description | The FDA Global Substance Registration System (GSRS) enables the efficient and accurate exchange of information on what substances are in regulated products. Instead of relying on names, which vary across regulatory domains, countries, and regions, the GSRS knowledge base makes it possible for substances to be defined by standardized, scientific descriptions. | |
Explanation | Unless otherwise noted, the contents of the FDA website (www.fda.gov), both text and graphics, are not copyrighted. They are in the public domain and may be republished, reprinted and otherwise used freely by anyone without the need to obtain permission from FDA. Credit to the U.S. Food and Drug Administration as the source is appreciated but not required. | |
Retrosynthesis Analysis
AI-Powered Synthesis Planning: Our tool employs the Template_relevance Pistachio, Template_relevance Bkms_metabolic, Template_relevance Pistachio_ringbreaker, Template_relevance Reaxys, Template_relevance Reaxys_biocatalysis model, leveraging a vast database of chemical reactions to predict feasible synthetic routes.
One-Step Synthesis Focus: Specifically designed for one-step synthesis, it provides concise and direct routes for your target compounds, streamlining the synthesis process.
Accurate Predictions: Utilizing the extensive PISTACHIO, BKMS_METABOLIC, PISTACHIO_RINGBREAKER, REAXYS, REAXYS_BIOCATALYSIS database, our tool offers high-accuracy predictions, reflecting the latest in chemical research and data.
Strategy Settings
Precursor scoring | Relevance Heuristic |
---|---|
Min. plausibility | 0.01 |
Model | Template_relevance |
Template Set | Pistachio/Bkms_metabolic/Pistachio_ringbreaker/Reaxys/Reaxys_biocatalysis |
Top-N result to add to graph | 6 |
Feasible Synthetic Routes
Q1: How does TCO-NHS ester contribute to the pretargeted imaging strategy described in the research?
A1: this compound plays a crucial role in the bioorthogonal click chemistry employed in this imaging strategy. The researchers first modify an antibody targeting the PD-L1 protein with this compound. This modification allows the antibody to conjugate with a Tetrazine-functionalized imaging agent through a rapid and highly specific Diels-Alder reaction in vivo. [] This two-step approach minimizes off-target effects and enhances the signal-to-noise ratio for improved tumor imaging.
Disclaimer and Information on In-Vitro Research Products
Please be aware that all articles and product information presented on BenchChem are intended solely for informational purposes. The products available for purchase on BenchChem are specifically designed for in-vitro studies, which are conducted outside of living organisms. In-vitro studies, derived from the Latin term "in glass," involve experiments performed in controlled laboratory settings using cells or tissues. It is important to note that these products are not categorized as medicines or drugs, and they have not received approval from the FDA for the prevention, treatment, or cure of any medical condition, ailment, or disease. We must emphasize that any form of bodily introduction of these products into humans or animals is strictly prohibited by law. It is essential to adhere to these guidelines to ensure compliance with legal and ethical standards in research and experimentation.