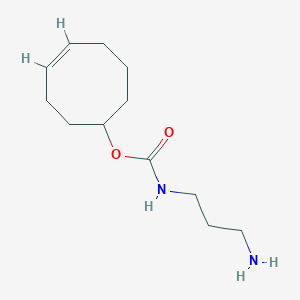
TCO-amine
Overview
Description
Mechanism of Action
Target of Action
The primary target of TCO-amine is indoleamine 2,3-dioxygenase (IDO1) . IDO1 is an enzyme that plays a crucial role in the metabolism of tryptophan, an essential amino acid, along the kynurenine pathway . This enzyme is often upregulated in various disease states, including cancer, making it an attractive target for therapeutic interventions .
Mode of Action
This compound interacts with its target, IDO1, through a process known as bioorthogonal chemistry . Specifically, this compound is designed to bind to IDO1, thereby inhibiting its activity . This interaction between this compound and IDO1 leads to changes in the metabolic processes regulated by IDO1, particularly the metabolism of tryptophan .
Biochemical Pathways
The interaction of this compound with IDO1 affects the kynurenine pathway , a major route of tryptophan catabolism . By inhibiting IDO1, this compound can potentially disrupt the production of kynurenine and other downstream metabolites, which have been implicated in immune regulation and various pathophysiological processes .
Pharmacokinetics
The ADME (Absorption, Distribution, Metabolism, and Excretion) properties of this compound are critical for its bioavailability and overall therapeutic efficacy . As a small molecule, this compound can be absorbed and distributed throughout the body. It may undergo metabolism to form various metabolites, some of which could also have biological activity . The excretion of this compound and its metabolites would eventually determine the duration of its action .
Result of Action
The molecular and cellular effects of this compound’s action primarily involve the modulation of IDO1 activity and, consequently, the kynurenine pathway . By inhibiting IDO1, this compound can alter the cellular concentrations of tryptophan and its metabolites, potentially affecting various cellular functions and immune responses .
Action Environment
Environmental factors, such as nutrition, can influence the action, efficacy, and stability of this compound . For instance, the availability of dietary tryptophan could affect the activity of IDO1 and, therefore, the efficacy of this compound . Moreover, the stability of this compound could be influenced by various factors, including pH and temperature .
Biochemical Analysis
Biochemical Properties
TCO-amine plays a crucial role in biochemical reactions, especially in the synthesis of PROTACs (proteolysis-targeting chimeras). It acts as a linker that connects two different ligands: one for an E3 ubiquitin ligase and the other for the target protein . This interaction exploits the intracellular ubiquitin-proteasome system to selectively degrade target proteins. This compound interacts with enzymes and proteins involved in the ubiquitin-proteasome pathway, facilitating the degradation of specific proteins .
Cellular Effects
This compound influences various cellular processes, including cell signaling pathways, gene expression, and cellular metabolism. Its role in PROTACs allows for the targeted degradation of proteins, which can modulate cell signaling pathways and alter gene expression patterns . This targeted degradation can lead to changes in cellular metabolism by removing key regulatory proteins, thereby affecting cellular functions .
Molecular Mechanism
At the molecular level, this compound exerts its effects through the inverse electron demand Diels-Alder (IEDDA) reaction with tetrazines . This reaction is one of the fastest bioorthogonal reactions known and is highly efficient under physiological conditions. This compound binds to tetrazine-functionalized biomolecules, forming a stable covalent bond. This interaction can lead to enzyme inhibition or activation, depending on the target protein, and can result in changes in gene expression .
Temporal Effects in Laboratory Settings
In laboratory settings, the effects of this compound can change over time. The compound is generally stable under recommended storage conditions, but its stability and degradation can vary depending on the experimental conditions . Long-term effects on cellular function have been observed in both in vitro and in vivo studies, where this compound has been shown to maintain its activity over extended periods .
Dosage Effects in Animal Models
The effects of this compound vary with different dosages in animal models. At lower doses, this compound can effectively target and degrade specific proteins without causing significant toxicity . At higher doses, toxic or adverse effects may be observed, including potential off-target effects and cellular toxicity . It is essential to determine the optimal dosage to achieve the desired therapeutic effects while minimizing adverse outcomes.
Metabolic Pathways
This compound is involved in metabolic pathways related to protein degradation and cellular signaling . It interacts with enzymes and cofactors in the ubiquitin-proteasome system, influencing metabolic flux and metabolite levels. The compound’s role in PROTACs highlights its importance in regulating protein turnover and maintaining cellular homeostasis .
Transport and Distribution
Within cells and tissues, this compound is transported and distributed through interactions with transporters and binding proteins . Its localization and accumulation can be influenced by these interactions, affecting its overall activity and function. The compound’s ability to form stable covalent bonds with target biomolecules ensures its effective distribution within the cellular environment .
Subcellular Localization
This compound’s subcellular localization is determined by its chemical structure and interactions with cellular components . It can be directed to specific compartments or organelles through targeting signals or post-translational modifications. This localization is crucial for its activity, as it ensures that this compound reaches its intended targets within the cell .
Preparation Methods
Synthetic Routes and Reaction Conditions
The synthesis of TCO-amine involves the functionalization of trans-cyclooctene with an amine group. This process can be carried out using a flow photoreactor, which allows for efficient and high-yield production .
Industrial Production Methods
Industrial production of this compound typically involves large-scale photoisomerization setups to convert cis-cyclooctene to trans-cyclooctene. The amine group is then introduced using standard coupling techniques such as DCC (dicyclohexylcarbodiimide) or EDC (1-ethyl-3-(3-dimethylaminopropyl)carbodiimide) in the presence of carboxyl-containing compounds .
Chemical Reactions Analysis
Types of Reactions
TCO-amine primarily undergoes bioorthogonal reactions, particularly the inverse electron demand Diels-Alder (IEDDA) reaction with tetrazines . This reaction is highly specific and occurs rapidly under mild conditions, making it suitable for in vivo applications .
Common Reagents and Conditions
The IEDDA reaction between this compound and tetrazines does not require a catalyst and proceeds efficiently at room temperature. The reaction forms a stable covalent bond, resulting in the formation of a dihydropyridazine intermediate, which can further eliminate a leaving group .
Major Products
The major product of the IEDDA reaction between this compound and tetrazines is a stable covalent adduct, which can be used for various applications in chemical biology and medicine .
Scientific Research Applications
TCO-amine has a wide range of applications in scientific research:
Comparison with Similar Compounds
TCO-amine is unique due to its high reactivity and specificity in the IEDDA reaction. Similar compounds include:
Vinylboronic acids: These compounds also react with tetrazines but have different reactivity profiles.
Cyclopropenes: Another class of compounds that can undergo bioorthogonal reactions with tetrazines.
This compound stands out due to its rapid reaction kinetics and stability under physiological conditions, making it highly suitable for in vivo applications.
Properties
IUPAC Name |
[(4Z)-cyclooct-4-en-1-yl] N-(3-aminopropyl)carbamate | |
---|---|---|
Source | PubChem | |
URL | https://pubchem.ncbi.nlm.nih.gov | |
Description | Data deposited in or computed by PubChem | |
InChI |
InChI=1S/C12H22N2O2/c13-9-6-10-14-12(15)16-11-7-4-2-1-3-5-8-11/h1-2,11H,3-10,13H2,(H,14,15)/b2-1- | |
Source | PubChem | |
URL | https://pubchem.ncbi.nlm.nih.gov | |
Description | Data deposited in or computed by PubChem | |
InChI Key |
MMNVKTBTNQJBEE-UPHRSURJSA-N | |
Source | PubChem | |
URL | https://pubchem.ncbi.nlm.nih.gov | |
Description | Data deposited in or computed by PubChem | |
Canonical SMILES |
C1CC=CCCC(C1)OC(=O)NCCCN | |
Source | PubChem | |
URL | https://pubchem.ncbi.nlm.nih.gov | |
Description | Data deposited in or computed by PubChem | |
Isomeric SMILES |
C1C/C=C\CCC(C1)OC(=O)NCCCN | |
Source | PubChem | |
URL | https://pubchem.ncbi.nlm.nih.gov | |
Description | Data deposited in or computed by PubChem | |
Molecular Formula |
C12H22N2O2 | |
Source | PubChem | |
URL | https://pubchem.ncbi.nlm.nih.gov | |
Description | Data deposited in or computed by PubChem | |
DSSTOX Substance ID |
DTXSID501175522 | |
Record name | Carbamic acid, N-(3-aminopropyl)-, (4E)-4-cycloocten-1-yl ester | |
Source | EPA DSSTox | |
URL | https://comptox.epa.gov/dashboard/DTXSID501175522 | |
Description | DSSTox provides a high quality public chemistry resource for supporting improved predictive toxicology. | |
Molecular Weight |
226.32 g/mol | |
Source | PubChem | |
URL | https://pubchem.ncbi.nlm.nih.gov | |
Description | Data deposited in or computed by PubChem | |
CAS No. |
1799962-26-7 | |
Record name | Carbamic acid, N-(3-aminopropyl)-, (4E)-4-cycloocten-1-yl ester | |
Source | CAS Common Chemistry | |
URL | https://commonchemistry.cas.org/detail?cas_rn=1799962-26-7 | |
Description | CAS Common Chemistry is an open community resource for accessing chemical information. Nearly 500,000 chemical substances from CAS REGISTRY cover areas of community interest, including common and frequently regulated chemicals, and those relevant to high school and undergraduate chemistry classes. This chemical information, curated by our expert scientists, is provided in alignment with our mission as a division of the American Chemical Society. | |
Explanation | The data from CAS Common Chemistry is provided under a CC-BY-NC 4.0 license, unless otherwise stated. | |
Record name | Carbamic acid, N-(3-aminopropyl)-, (4E)-4-cycloocten-1-yl ester | |
Source | EPA DSSTox | |
URL | https://comptox.epa.gov/dashboard/DTXSID501175522 | |
Description | DSSTox provides a high quality public chemistry resource for supporting improved predictive toxicology. | |
Retrosynthesis Analysis
AI-Powered Synthesis Planning: Our tool employs the Template_relevance Pistachio, Template_relevance Bkms_metabolic, Template_relevance Pistachio_ringbreaker, Template_relevance Reaxys, Template_relevance Reaxys_biocatalysis model, leveraging a vast database of chemical reactions to predict feasible synthetic routes.
One-Step Synthesis Focus: Specifically designed for one-step synthesis, it provides concise and direct routes for your target compounds, streamlining the synthesis process.
Accurate Predictions: Utilizing the extensive PISTACHIO, BKMS_METABOLIC, PISTACHIO_RINGBREAKER, REAXYS, REAXYS_BIOCATALYSIS database, our tool offers high-accuracy predictions, reflecting the latest in chemical research and data.
Strategy Settings
Precursor scoring | Relevance Heuristic |
---|---|
Min. plausibility | 0.01 |
Model | Template_relevance |
Template Set | Pistachio/Bkms_metabolic/Pistachio_ringbreaker/Reaxys/Reaxys_biocatalysis |
Top-N result to add to graph | 6 |
Feasible Synthetic Routes
Disclaimer and Information on In-Vitro Research Products
Please be aware that all articles and product information presented on BenchChem are intended solely for informational purposes. The products available for purchase on BenchChem are specifically designed for in-vitro studies, which are conducted outside of living organisms. In-vitro studies, derived from the Latin term "in glass," involve experiments performed in controlled laboratory settings using cells or tissues. It is important to note that these products are not categorized as medicines or drugs, and they have not received approval from the FDA for the prevention, treatment, or cure of any medical condition, ailment, or disease. We must emphasize that any form of bodily introduction of these products into humans or animals is strictly prohibited by law. It is essential to adhere to these guidelines to ensure compliance with legal and ethical standards in research and experimentation.