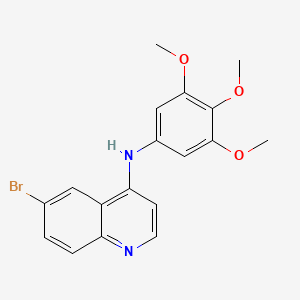
Sgc-gak-1
- Click on QUICK INQUIRY to receive a quote from our team of experts.
- With the quality product at a COMPETITIVE price, you can focus more on your research.
Overview
Description
SGC-GAK-1 is an inhibitor of cyclin G-associated kinase (GAK; Ki = 3.1 nM). It is greater than 50-fold selective for GAK over a panel of 400 kinases at 1 µM but does bind to RIPK2 in a bioluminescence resonance energy transfer (BRET) engagement assay (IC50 = 360 nM). SCG-GAK-1 reduces the viability of LNCaP and 22Rv1 prostate cancer cells with IC50 values of 0.65 and 0.17 µM, respectively. It also inhibits dengue virus infection in Huh7 cells (EC50 = 0.08 µM).
SGC GAK 1 is a high affinity cyclin G associated kinase (GAK) inhibitor.
Mechanism of Action
Target of Action
The primary target of Sgc-gak-1 is the Cyclin G-associated kinase (GAK) . GAK is a 160 kDa serine/threonine kinase and is a member of the numb-associated kinase (NAK) family . It plays a crucial role in various biological processes, including membrane trafficking, protein sorting, and centrosome maturation . It is also involved in neuronal development, making it a potential therapeutic target for neurodevelopmental disorders .
Mode of Action
This compound acts as a potent inhibitor of GAK by targeting its ATP-binding site . It selectively binds to GAK, reducing its function . This results in decreased neurite outgrowth and synaptogenesis, as evidenced by reduced neurite length and decreased synapse number .
Biochemical Pathways
The inhibition of GAK by this compound affects the protein phosphorylation process, an important modification in signal transduction pathways . Specifically, it decreases the phosphorylation of neurofilament 200-kDa subunits . This suggests that GAK may be involved in the regulation of neurite outgrowth and synaptogenesis, which are critical processes in neuronal development .
Pharmacokinetics
This rapid metabolism resulted in quick clearance in liver microsomes and in mice, limiting its utility in in vivo studies .
Result of Action
The inhibition of GAK by this compound leads to impaired neurite outgrowth and synaptogenesis . This is manifested as reduced neurite length and decreased synapse number in cultured neurons . These results suggest that GAK may be physiologically involved in normal neuronal development, and that decreased GAK function may be related to neurodevelopmental disorders .
Action Environment
The action of this compound can be influenced by environmental factors such as the presence of other kinases. For instance, while this compound is highly selective in a kinome-wide screen, cellular engagement assays have identified receptor-interacting protein kinase 2 (RIPK2) as a collateral target . Additionally, the metabolic stability of this compound can be affected by the presence of cytochrome P450 enzymes in the liver .
Biochemical Analysis
Biochemical Properties
Sgc-gak-1 interacts with GAK, a 160 kDa serine/threonine kinase originally identified as a direct association partner with cyclin G . The compound has a high affinity for GAK, with a dissociation constant (K D) of 1.9 nM .
Cellular Effects
This compound has multiple biological roles and disease associations. It is involved in membrane trafficking and sorting of proteins, including as an essential cofactor for HSC70-dependent uncoating of clathrin coated vesicles in the cytoplasm . This compound is required for maintenance of centrosome maturation and progression through mitosis .
Molecular Mechanism
This compound exerts its effects at the molecular level by targeting the ATP-binding site of GAK . It is highly selective in a kinome-wide screen .
Temporal Effects in Laboratory Settings
It is known that this compound increases the thermal stability of the isolated GAK kinase domain .
Metabolic Pathways
This compound is involved in the metabolic pathway of GAK, a member of the numb-associated kinase (NAK) family .
Transport and Distribution
Within the cell, this compound localizes to the Golgi complex, cytoplasm, and nucleus .
Subcellular Localization
This compound is localized within the Golgi complex, cytoplasm, and nucleus
Properties
IUPAC Name |
6-bromo-N-(3,4,5-trimethoxyphenyl)quinolin-4-amine |
Source
|
---|---|---|
Source | PubChem | |
URL | https://pubchem.ncbi.nlm.nih.gov | |
Description | Data deposited in or computed by PubChem | |
InChI |
InChI=1S/C18H17BrN2O3/c1-22-16-9-12(10-17(23-2)18(16)24-3)21-15-6-7-20-14-5-4-11(19)8-13(14)15/h4-10H,1-3H3,(H,20,21) |
Source
|
Source | PubChem | |
URL | https://pubchem.ncbi.nlm.nih.gov | |
Description | Data deposited in or computed by PubChem | |
InChI Key |
AUOSKLDNVNGKRR-UHFFFAOYSA-N |
Source
|
Source | PubChem | |
URL | https://pubchem.ncbi.nlm.nih.gov | |
Description | Data deposited in or computed by PubChem | |
Canonical SMILES |
COC1=CC(=CC(=C1OC)OC)NC2=C3C=C(C=CC3=NC=C2)Br |
Source
|
Source | PubChem | |
URL | https://pubchem.ncbi.nlm.nih.gov | |
Description | Data deposited in or computed by PubChem | |
Molecular Formula |
C18H17BrN2O3 |
Source
|
Source | PubChem | |
URL | https://pubchem.ncbi.nlm.nih.gov | |
Description | Data deposited in or computed by PubChem | |
Molecular Weight |
389.2 g/mol |
Source
|
Source | PubChem | |
URL | https://pubchem.ncbi.nlm.nih.gov | |
Description | Data deposited in or computed by PubChem | |
Q1: What makes SGC-GAK-1 a valuable tool for studying cyclin G associated kinase (GAK)?
A1: this compound stands out as a potent and selective inhibitor of GAK, making it a valuable chemical probe for investigating the biological functions of this understudied kinase. [, ] Unlike many other kinase inhibitors, this compound demonstrates high selectivity for GAK, with limited activity against other kinases except for RIPK2. [] This selectivity is crucial for attributing observed cellular effects specifically to GAK inhibition. Researchers have successfully used this compound to demonstrate the role of GAK in neurite outgrowth and synapse formation, highlighting its utility in dissecting GAK's involvement in various cellular processes. []
Q2: Does this compound have any off-target effects?
A2: While highly selective for GAK, this compound has been found to also inhibit RIPK2, albeit to a lesser extent. [] This finding highlights the importance of using appropriate controls in experiments involving this compound. To address this, researchers have identified a structurally related molecule, SGC-GAK-1N, which exhibits significantly lower potency towards GAK but retains activity against RIPK2. [] Additionally, a compound known as HY-19764 demonstrates potent RIPK2 inhibition without affecting GAK. [] Utilizing these control compounds alongside this compound allows researchers to differentiate between the effects of GAK inhibition and potential off-target effects mediated through RIPK2.
Q3: What future directions are being pursued in the development of GAK inhibitors?
A4: Research efforts continue to focus on developing an optimized in vivo probe for GAK. While this compound has proven valuable for in vitro studies, its rapid metabolism necessitates further optimization for in vivo applications. [] Current research explores two main avenues: (1) designing novel GAK inhibitors with improved metabolic stability while maintaining high potency and selectivity, and (2) utilizing co-administration strategies with cytochrome P450 inhibitors to enhance the in vivo exposure of existing inhibitors like this compound. [, ] These efforts aim to provide researchers with robust tools to fully elucidate the therapeutic potential of GAK inhibition.
Disclaimer and Information on In-Vitro Research Products
Please be aware that all articles and product information presented on BenchChem are intended solely for informational purposes. The products available for purchase on BenchChem are specifically designed for in-vitro studies, which are conducted outside of living organisms. In-vitro studies, derived from the Latin term "in glass," involve experiments performed in controlled laboratory settings using cells or tissues. It is important to note that these products are not categorized as medicines or drugs, and they have not received approval from the FDA for the prevention, treatment, or cure of any medical condition, ailment, or disease. We must emphasize that any form of bodily introduction of these products into humans or animals is strictly prohibited by law. It is essential to adhere to these guidelines to ensure compliance with legal and ethical standards in research and experimentation.