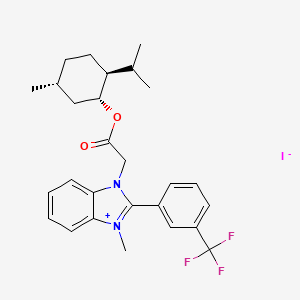
S-Gboxin
Overview
Description
S-Gboxin is a small molecule that functions as an inhibitor of oxidative phosphorylation (OXPHOS). It is an active analogue of Gboxin and has shown potent antitumor activity, particularly against glioblastoma, a type of brain cancer. This compound inhibits the growth of both mouse and human glioblastoma cells by targeting the mitochondrial oxidative phosphorylation pathway .
Mechanism of Action
Target of Action
S-Gboxin, a functional analogue of Gboxin, is a small molecule that specifically inhibits the growth of primary mouse and human glioblastoma cells . The primary target of this compound is the oxidative phosphorylation (OXPHOS) complexes located in the mitochondria of cancer cells . These complexes play a crucial role in energy production within the cell.
Mode of Action
This compound operates by associating with mitochondrial OXPHOS complexes in a manner dependent on the proton gradient of the inner mitochondrial membrane . It relies on its positive charge to interact with these complexes . Specifically, it inhibits the activity of F0F1 ATP synthase, a key enzyme in the OXPHOS pathway .
Biochemical Pathways
The primary biochemical pathway affected by this compound is the OXPHOS pathway. This pathway is responsible for the majority of ATP production in cells, particularly in cancer cells that have a high metabolic rate . By inhibiting the F0F1 ATP synthase, this compound disrupts the OXPHOS pathway, leading to a decrease in ATP production and thus affecting the energy metabolism of the cancer cells .
Result of Action
The primary result of this compound’s action is the rapid and irreversible compromise of oxygen consumption in glioblastoma cells . This leads to a decrease in ATP production, affecting the energy metabolism of the cells and ultimately leading to cell death . In animal models, administration of a metabolically stable this compound analogue has been shown to inhibit glioblastoma growth .
Action Environment
The action of this compound is influenced by the unique metabolic environment of cancer cells. Specifically, this compound is more effective in cells with a high rate of OXPHOS, such as cancer cells . Furthermore, the effectiveness of this compound is also influenced by the status of the mitochondrial permeability transition pore (mPTP) in the cells . Cells with an inactive mPTP are more sensitive to this compound, as the inactive mPTP leads to a higher proton gradient and pH within the mitochondrial matrix, promoting the accumulation of this compound .
Biochemical Analysis
Biochemical Properties
S-Gboxin is an oxidative phosphorylation inhibitor that targets glioblastoma . It relies on its positive charge to associate with mitochondrial oxidative phosphorylation complexes in a manner that is dependent on the proton gradient of the inner mitochondrial membrane . It inhibits the activity of F0F1 ATP synthase .
Cellular Effects
This compound rapidly and irreversibly compromises oxygen consumption in glioblastoma cells . It specifically inhibits the growth of primary mouse and human glioblastoma cells but not that of mouse embryonic fibroblasts or neonatal astrocytes .
Molecular Mechanism
This compound exerts its effects at the molecular level by associating with mitochondrial oxidative phosphorylation complexes . It inhibits the activity of F0F1 ATP synthase, a key enzyme in the process of oxidative phosphorylation .
Temporal Effects in Laboratory Settings
The effects of this compound on glioblastoma cells are rapid and irreversible . It quickly compromises oxygen consumption in these cells, leading to their death .
Dosage Effects in Animal Models
Administration of a metabolically stable this compound analogue inhibits glioblastoma allografts and patient-derived xenografts . The exact dosage effects in animal models are not mentioned in the available literature.
Metabolic Pathways
This compound is involved in the metabolic pathway of oxidative phosphorylation . It inhibits the activity of F0F1 ATP synthase, a key enzyme in this pathway .
Transport and Distribution
This compound associates with mitochondrial oxidative phosphorylation complexes in a manner that is dependent on the proton gradient of the inner mitochondrial membrane . This suggests that it is transported to the mitochondria where it exerts its effects.
Subcellular Localization
This compound is localized in the mitochondria of glioblastoma cells . It associates with mitochondrial oxidative phosphorylation complexes to exert its effects .
Preparation Methods
The preparation of S-Gboxin involves several synthetic steps. The process begins with the reaction of o-phenylenediamine with N-propionaldehyde to form 2-ethyl-1H-benzimidazole. This intermediate undergoes N-alkylation with chloroacetic acid-L-menthyl ester to generate 2-(2-ethyl-1H-benzimidazole) acetic acid-L-menthyl ester. Methylation using methyl iodide produces benzimidazolium salt, which is then subjected to ion exchange to obtain the final product, this compound . The method is characterized by mild reaction conditions, high purity, and high yield, making it suitable for industrial production.
Chemical Reactions Analysis
S-Gboxin undergoes various chemical reactions, including:
Oxidation: this compound can be oxidized under specific conditions, leading to the formation of oxidized derivatives.
Reduction: The compound can be reduced to yield reduced forms, although this is less common.
Substitution: this compound can participate in substitution reactions, where functional groups are replaced by other groups.
Common Reagents and Conditions: Typical reagents include oxidizing agents like hydrogen peroxide and reducing agents like sodium borohydride. Reaction conditions often involve controlled temperatures and pH levels.
Major Products: The major products formed from these reactions depend on the specific reagents and conditions used. .
Scientific Research Applications
S-Gboxin has a wide range of scientific research applications:
Chemistry: It is used as a tool to study oxidative phosphorylation and mitochondrial function.
Biology: this compound is employed in research on cancer cell metabolism, particularly in glioblastoma.
Medicine: The compound has shown promise as a potential therapeutic agent for glioblastoma due to its ability to inhibit tumor growth.
Industry: This compound is used in the development of antitumor drugs and in the study of mitochondrial inhibitors
Comparison with Similar Compounds
S-Gboxin is compared with other similar compounds, such as Gboxin and other OXPHOS inhibitors:
Gboxin: S-Gboxin is an active analogue of Gboxin with enhanced metabolic stability and pharmacokinetic properties.
Other OXPHOS Inhibitors: Compounds like FCCP and IACS-010759 also inhibit oxidative phosphorylation but differ in their specific targets and mechanisms of action.
Conclusion
S-Gboxin is a potent inhibitor of oxidative phosphorylation with significant antitumor activity, particularly against glioblastoma. Its unique mechanism of action and enhanced stability make it a valuable tool in scientific research and a promising candidate for therapeutic development.
Properties
IUPAC Name |
[(1R,2S,5R)-5-methyl-2-propan-2-ylcyclohexyl] 2-[3-methyl-2-[3-(trifluoromethyl)phenyl]benzimidazol-3-ium-1-yl]acetate;iodide | |
---|---|---|
Source | PubChem | |
URL | https://pubchem.ncbi.nlm.nih.gov | |
Description | Data deposited in or computed by PubChem | |
InChI |
InChI=1S/C27H32F3N2O2.HI/c1-17(2)21-13-12-18(3)14-24(21)34-25(33)16-32-23-11-6-5-10-22(23)31(4)26(32)19-8-7-9-20(15-19)27(28,29)30;/h5-11,15,17-18,21,24H,12-14,16H2,1-4H3;1H/q+1;/p-1/t18-,21+,24-;/m1./s1 | |
Source | PubChem | |
URL | https://pubchem.ncbi.nlm.nih.gov | |
Description | Data deposited in or computed by PubChem | |
InChI Key |
DCAJNAWCJSUZDG-DZJKTSMVSA-M | |
Source | PubChem | |
URL | https://pubchem.ncbi.nlm.nih.gov | |
Description | Data deposited in or computed by PubChem | |
Canonical SMILES |
CC1CCC(C(C1)OC(=O)CN2C3=CC=CC=C3[N+](=C2C4=CC(=CC=C4)C(F)(F)F)C)C(C)C.[I-] | |
Source | PubChem | |
URL | https://pubchem.ncbi.nlm.nih.gov | |
Description | Data deposited in or computed by PubChem | |
Isomeric SMILES |
C[C@@H]1CC[C@H]([C@@H](C1)OC(=O)CN2C3=CC=CC=C3[N+](=C2C4=CC(=CC=C4)C(F)(F)F)C)C(C)C.[I-] | |
Source | PubChem | |
URL | https://pubchem.ncbi.nlm.nih.gov | |
Description | Data deposited in or computed by PubChem | |
Molecular Formula |
C27H32F3IN2O2 | |
Source | PubChem | |
URL | https://pubchem.ncbi.nlm.nih.gov | |
Description | Data deposited in or computed by PubChem | |
Molecular Weight |
600.5 g/mol | |
Source | PubChem | |
URL | https://pubchem.ncbi.nlm.nih.gov | |
Description | Data deposited in or computed by PubChem | |
CAS No. |
2101317-21-7 | |
Record name | 1H-Benzimidazolium, 1-methyl-3-[2-[[(1R,2S,5R)-5-methyl-2-(1-methylethyl)cyclohexyl]oxy]-2-oxoethyl]-2-[3-(trifluoromethyl)phenyl]-, iodide (1:1) | |
Source | CAS Common Chemistry | |
URL | https://commonchemistry.cas.org/detail?cas_rn=2101317-21-7 | |
Description | CAS Common Chemistry is an open community resource for accessing chemical information. Nearly 500,000 chemical substances from CAS REGISTRY cover areas of community interest, including common and frequently regulated chemicals, and those relevant to high school and undergraduate chemistry classes. This chemical information, curated by our expert scientists, is provided in alignment with our mission as a division of the American Chemical Society. | |
Explanation | The data from CAS Common Chemistry is provided under a CC-BY-NC 4.0 license, unless otherwise stated. | |
Q1: The research article highlights the importance of β-catenin-IRP2-primed iron availability in cancer cells. Could you elaborate on the mechanism by which this pathway contributes to cancer progression?
A1: The research paper you cited [] focuses on the role of β-catenin in regulating iron metabolism in cancer cells and its potential as a therapeutic target. While the study doesn't explicitly investigate a compound called "S-Gboxin," it suggests that disrupting the β-catenin-IRP2 axis could be a promising strategy for cancer treatment.
Disclaimer and Information on In-Vitro Research Products
Please be aware that all articles and product information presented on BenchChem are intended solely for informational purposes. The products available for purchase on BenchChem are specifically designed for in-vitro studies, which are conducted outside of living organisms. In-vitro studies, derived from the Latin term "in glass," involve experiments performed in controlled laboratory settings using cells or tissues. It is important to note that these products are not categorized as medicines or drugs, and they have not received approval from the FDA for the prevention, treatment, or cure of any medical condition, ailment, or disease. We must emphasize that any form of bodily introduction of these products into humans or animals is strictly prohibited by law. It is essential to adhere to these guidelines to ensure compliance with legal and ethical standards in research and experimentation.