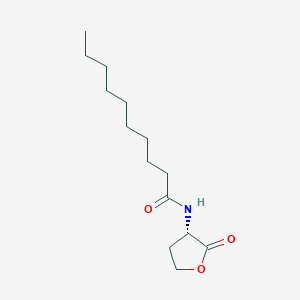
N-Decanoyl-L-Homoserine lactone
Overview
Description
N-Decanoyl-L-homoserine lactone (C10-HSL) is a member of the N-acyl-L-homoserine lactone (AHL) family, a class of quorum sensing (QS) signaling molecules produced by Gram-negative bacteria. These molecules regulate gene expression in response to population density, coordinating behaviors such as biofilm formation, virulence factor secretion, and symbiosis . Its structure is pivotal in determining its biological activity, solubility, and interaction with microbial or eukaryotic systems .
Preparation Methods
Synthetic Routes:: C10-HSL can be synthesized through various methods, including chemical synthesis and enzymatic routes. One common approach involves the condensation of decanoic acid (decanoate) with L-homoserine lactone. The reaction typically occurs under mild conditions using coupling agents or catalysts.
Industrial Production:: While industrial-scale production methods for C10-HSL are less common, research efforts have focused on optimizing microbial fermentation processes. Engineered bacterial strains can produce AHLs, including C10-HSL, as part of their metabolic pathways.
Chemical Reactions Analysis
Reactions:: C10-HSL can participate in several chemical reactions:
Hydrolysis: Under acidic or basic conditions, C10-HSL can undergo hydrolysis to yield decanoic acid and L-homoserine lactone.
Oxidation: Oxidative processes can lead to the formation of various oxidation products.
Substitution: C10-HSL can react with nucleophiles, such as amines or thiols, resulting in substitution products.
Hydrolysis: Acidic or basic aqueous solutions.
Oxidation: Oxidizing agents (e.g., hydrogen peroxide, potassium permanganate).
Substitution: Nucleophilic reagents (e.g., ammonia, mercaptans).
Major Products:: The major products depend on the specific reaction conditions. Hydrolysis yields decanoic acid and L-homoserine lactone, while oxidation and substitution reactions lead to various derivatives.
Scientific Research Applications
Microbial Communication and Biofilm Formation
Quorum Sensing Inhibition
C10-HSL is significant in the study of quorum sensing as it regulates gene expression in Gram-negative bacteria such as Escherichia coli and Salmonella. Researchers have focused on developing inhibitors that target AHL signaling to disrupt biofilm formation, which is critical in preventing chronic infections. For instance, studies have shown that synthetic AHLs can inhibit the growth of pathogenic bacteria like Pseudomonas aeruginosa by interfering with their QS mechanisms .
Case Study: Biofilm Disruption
A study demonstrated that the use of AHL lactonases could effectively degrade C10-HSL, leading to reduced biofilm formation in Pseudomonas aeruginosa. This approach has potential therapeutic applications in treating infections associated with biofilms .
Agricultural Applications
Plant Growth Regulation
C10-HSL has been investigated for its effects on plant growth. Research indicates that it can stimulate adventitious root formation in plants like Vigna radiata (mung bean). The compound enhances auxin signaling pathways, promoting root development .
Compound | Effect on Plant | Mechanism |
---|---|---|
N-Decanoyl-L-Homoserine Lactone | Accelerates root formation | Enhances auxin response genes |
Antiviral and Anticancer Properties
Regulation of Virulence Factors
In addition to its role in microbial communication, C10-HSL has been shown to affect virulence factors in various pathogens. Studies indicate that it can regulate exoprotease production in Pseudomonas aeruginosa, which is linked to its pathogenicity .
Antiproliferative Effects
Recent findings suggest that C10-HSL exhibits antiproliferative effects against human oral squamous carcinoma cells. The compound influences cancer cell behavior, indicating potential therapeutic avenues for cancer treatment .
Biochemical Mechanisms
Signal Transduction Pathways
C10-HSL operates through specific receptors in target organisms, triggering signal transduction pathways that affect gene expression and cellular responses. For example, it has been shown to activate nitric oxide production in plants, which is crucial for various physiological processes .
Mechanism of Action
C10-HSL’s effects are mediated through specific receptors in bacterial cells. Upon reaching a critical concentration, it activates gene expression, influencing bacterial behavior and community dynamics. The exact molecular targets and pathways vary among different bacterial species.
Comparison with Similar Compounds
Structural and Functional Comparison with Key Analogues
Structural Features
AHLs vary in acyl chain length (C4–C16) and the presence of substituents (e.g., 3-oxo or 3-hydroxy groups). Key analogues of C10-HSL include:
Key Observations :
- Chain Length : Longer chains (e.g., C12-HSL) enhance hydrophobicity, affecting membrane permeability and receptor binding .
- Substituents: 3-Oxo groups (e.g., 3OC12-HSL) increase receptor affinity in P. aeruginosa QS systems, while 3-hydroxy groups reduce activity .
Quorum Sensing Modulation
- C10-HSL: Inhibits Chromobacterium violaceum violacein production at 100 nM (51% inhibition) . Induces biofilm formation in Vibrio alginolyticus at 10 µM .
- 3OC12-HSL: Potent activator of P. aeruginosa virulence pathways (e.g., elastase production) at 100 nM . Triggers apoptosis in mammalian cells (40% at 100 µM) .
- C6-HSL: Promotes Microcystis aeruginosa proliferation (138% increase) at 1 µM .
Plant-Microbe Interactions
- C10-HSL : Enhances tomato resistance to Botrytis cinerea (60 µM reduces lesion area by 50%) by upregulating antioxidant pathways .
- 3OC6-HSL : Induces systemic resistance in Arabidopsis via protein expression linked to oxidative stress .
Eukaryotic Effects
Detection and Analytical Methods
Biological Activity
N-Decanoyl-L-homoserine lactone (C10-HSL) is a member of the N-acyl homoserine lactones (AHLs) family, which are crucial signaling molecules in quorum sensing among Gram-negative bacteria. This article explores the biological activities of C10-HSL, focusing on its roles in bacterial communication, plant interactions, and potential applications in biotechnology and agriculture.
- Molecular Formula: C14H25NO3
- Molecular Weight: 255.353 g/mol
- Melting Point: 127-130 °C
- Density: 1.0 ± 0.1 g/cm³
- Boiling Point: 466.1 ± 34.0 °C at 760 mmHg
- Flash Point: 235.7 ± 25.7 °C
Quorum Sensing in Bacteria
C10-HSL plays a pivotal role in the quorum sensing mechanism, which enables bacteria to communicate and coordinate group behaviors based on population density. This signaling affects various physiological processes, including virulence factor production, biofilm formation, and bioluminescence in species such as Pseudomonas aeruginosa and Vibrio fischeri.
- Gene Regulation : C10-HSL regulates gene expression related to virulence and biofilm formation in pathogenic bacteria. For instance, it influences the production of exoproteases and other virulence factors in Erwinia carotovora and Burkholderia vietnamiensis .
- Inhibition of Pathogen Growth : Research indicates that C10-HSL can inhibit the growth of certain pathogens by modulating the expression of specific genes involved in defense mechanisms .
Effects on Plant Growth
Recent studies have highlighted the impact of AHLs, including C10-HSL, on plant growth and development:
- Root Growth Inhibition : C10-HSL has been shown to inhibit primary root growth in Arabidopsis thaliana. The underlying mechanisms involve alterations in auxin signaling pathways, which are critical for root architecture .
- Defense Responses : In plants like Medicago truncatula, exposure to AHLs leads to systemic accumulation of defense-related gene transcripts, enhancing resistance against biotrophic pathogens .
Study on Arabidopsis
A study investigating the effects of C10-HSL on Arabidopsis revealed that it inhibits primary root growth through interference with auxin signaling pathways. The research utilized pharmacological and genetic approaches to elucidate the signaling components involved .
Influence on Agricultural Crops
Research conducted on barley (Hordeum vulgare) and tropical legumes demonstrated that C10-HSL affects enzymatic activities related to antioxidative stress responses. These findings suggest potential applications for AHLs in enhancing crop resilience against environmental stressors .
Summary of Findings
Biological Activity | Effect | Organism/Model |
---|---|---|
Quorum Sensing | Regulates gene expression for virulence | Pseudomonas aeruginosa |
Root Growth Inhibition | Inhibits primary root growth | Arabidopsis thaliana |
Defense Response Activation | Enhances resistance against pathogens | Medicago truncatula |
Enzymatic Activity Modulation | Influences antioxidative enzyme activities | Hordeum vulgare, legume crops |
Q & A
Basic Research Questions
Q. What methodologies are commonly used to detect and quantify C10-HSL in bacterial cultures?
Thin-layer chromatography (TLC) coupled with biosensor strains (e.g., Agrobacterium tumefaciens with lacZ reporters) is a classical method for separating and identifying AHLs like C10-HSL. This approach distinguishes structural variants based on mobility and spot shape . Advanced techniques like LC-MS/MS provide higher sensitivity and specificity, enabling simultaneous quantification of multiple AHLs, including 3-hydroxy and 3-oxo derivatives .
Q. Which bacterial species produce C10-HSL as a quorum-sensing (QS) signal?
C10-HSL is associated with Pseudomonas fluorescens (as a 3-hydroxy derivative) and Aeromonas sobria, where it regulates virulence factors such as biofilm formation and extracellular protease production . Other Proteobacteria, including Burkholderia and Vibrio species, may also utilize structurally similar AHLs .
Q. How does C10-HSL structurally differ from other AHLs, and why does this matter?
C10-HSL has a 10-carbon acyl chain. Substitutions at the C3 position (e.g., 3-oxo, 3-hydroxy, or unmodified) influence its biological activity and detection. For example, 3-hydroxy-C10-HSL migrates differently on TLC than 3-oxo variants, complicating structural identification without mass spectrometry validation .
Advanced Research Questions
Q. How can conflicting data on QS-regulated behaviors be resolved when studying C10-HSL?
Discrepancies may arise from strain-specific regulatory networks or experimental conditions (e.g., nutrient availability). For instance, Aeromonas sobria biofilm formation is C10-HSL-dependent , but in Pseudomonas aeruginosa, longer-chain AHLs (e.g., 3-oxo-C12-HSL) dominate. Cross-validation using genetic knockouts (e.g., luxI mutants) and controlled induction experiments is critical .
Q. What molecular tools are available to study C10-HSL signaling in plant-bacteria interactions?
In Vigna radiata (mung bean), 3-oxo-C10-HSL induces adventitious roots via H2O2 and NO-dependent cGMP pathways. Researchers can use scavengers (e.g., catalase for H2O2) and inhibitors (e.g., L-NAME for NO synthase) to dissect these pathways. Auxin-response genes (e.g., AUX/IAA) serve as molecular markers .
Q. How can enzyme engineering improve specificity for C10-HSL in biotechnological applications?
Rational mutagenesis of penicillin G acylase (PGA) has created variants like YAF, which preferentially hydrolyze longer-chain AHLs (e.g., C10-HSL) over shorter ones. Structural dynamics of the enzyme's binding pocket can be optimized using computational modeling and directed evolution .
Q. What ecological roles do C10-HSL-degrading bacteria play?
Variovorax paradoxus metabolizes C10-HSL as a sole nitrogen and energy source, cleaving the homoserine lactone ring. This degradation impacts QS-mediated virulence in co-cultured pathogens. Studies using radiolabeled C10-HSL and knockout mutants can elucidate degradation pathways .
Properties
IUPAC Name |
N-[(3S)-2-oxooxolan-3-yl]decanamide | |
---|---|---|
Source | PubChem | |
URL | https://pubchem.ncbi.nlm.nih.gov | |
Description | Data deposited in or computed by PubChem | |
InChI |
InChI=1S/C14H25NO3/c1-2-3-4-5-6-7-8-9-13(16)15-12-10-11-18-14(12)17/h12H,2-11H2,1H3,(H,15,16)/t12-/m0/s1 | |
Source | PubChem | |
URL | https://pubchem.ncbi.nlm.nih.gov | |
Description | Data deposited in or computed by PubChem | |
InChI Key |
TZWZKDULKILUPV-LBPRGKRZSA-N | |
Source | PubChem | |
URL | https://pubchem.ncbi.nlm.nih.gov | |
Description | Data deposited in or computed by PubChem | |
Canonical SMILES |
CCCCCCCCCC(=O)NC1CCOC1=O | |
Source | PubChem | |
URL | https://pubchem.ncbi.nlm.nih.gov | |
Description | Data deposited in or computed by PubChem | |
Isomeric SMILES |
CCCCCCCCCC(=O)N[C@H]1CCOC1=O | |
Source | PubChem | |
URL | https://pubchem.ncbi.nlm.nih.gov | |
Description | Data deposited in or computed by PubChem | |
Molecular Formula |
C14H25NO3 | |
Source | PubChem | |
URL | https://pubchem.ncbi.nlm.nih.gov | |
Description | Data deposited in or computed by PubChem | |
DSSTOX Substance ID |
DTXSID00436062 | |
Record name | N-[(3S)-2-oxooxolan-3-yl]decanamide | |
Source | EPA DSSTox | |
URL | https://comptox.epa.gov/dashboard/DTXSID00436062 | |
Description | DSSTox provides a high quality public chemistry resource for supporting improved predictive toxicology. | |
Molecular Weight |
255.35 g/mol | |
Source | PubChem | |
URL | https://pubchem.ncbi.nlm.nih.gov | |
Description | Data deposited in or computed by PubChem | |
CAS No. |
177315-87-6 | |
Record name | N-[(3S)-2-oxooxolan-3-yl]decanamide | |
Source | EPA DSSTox | |
URL | https://comptox.epa.gov/dashboard/DTXSID00436062 | |
Description | DSSTox provides a high quality public chemistry resource for supporting improved predictive toxicology. | |
Retrosynthesis Analysis
AI-Powered Synthesis Planning: Our tool employs the Template_relevance Pistachio, Template_relevance Bkms_metabolic, Template_relevance Pistachio_ringbreaker, Template_relevance Reaxys, Template_relevance Reaxys_biocatalysis model, leveraging a vast database of chemical reactions to predict feasible synthetic routes.
One-Step Synthesis Focus: Specifically designed for one-step synthesis, it provides concise and direct routes for your target compounds, streamlining the synthesis process.
Accurate Predictions: Utilizing the extensive PISTACHIO, BKMS_METABOLIC, PISTACHIO_RINGBREAKER, REAXYS, REAXYS_BIOCATALYSIS database, our tool offers high-accuracy predictions, reflecting the latest in chemical research and data.
Strategy Settings
Precursor scoring | Relevance Heuristic |
---|---|
Min. plausibility | 0.01 |
Model | Template_relevance |
Template Set | Pistachio/Bkms_metabolic/Pistachio_ringbreaker/Reaxys/Reaxys_biocatalysis |
Top-N result to add to graph | 6 |
Feasible Synthetic Routes
Disclaimer and Information on In-Vitro Research Products
Please be aware that all articles and product information presented on BenchChem are intended solely for informational purposes. The products available for purchase on BenchChem are specifically designed for in-vitro studies, which are conducted outside of living organisms. In-vitro studies, derived from the Latin term "in glass," involve experiments performed in controlled laboratory settings using cells or tissues. It is important to note that these products are not categorized as medicines or drugs, and they have not received approval from the FDA for the prevention, treatment, or cure of any medical condition, ailment, or disease. We must emphasize that any form of bodily introduction of these products into humans or animals is strictly prohibited by law. It is essential to adhere to these guidelines to ensure compliance with legal and ethical standards in research and experimentation.