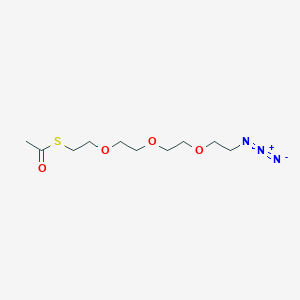
S-Acetyl-PEG3-azide
Overview
Description
S-Acetyl-PEG3-azide is a polyethylene glycol (PEG) derivative that contains an azide group and a sulfur acetyl group. The azide group is particularly useful for click chemistry, a class of biocompatible chemical reactions that are used to quickly and reliably join molecules together. The hydrophilic PEG spacer increases the solubility of the compound in aqueous media, making it highly versatile for various applications .
Preparation Methods
Synthetic Routes and Reaction Conditions
The synthesis of S-Acetyl-PEG3-azide typically involves the reaction of a PEG derivative with an azide group. One common method is the nucleophilic substitution reaction where an azide ion (N₃⁻) displaces a leaving group on the PEG derivative. This reaction is usually carried out in a polar aprotic solvent such as acetonitrile or dimethyl sulfoxide (DMSO) to enhance the nucleophilicity of the azide ion .
Industrial Production Methods
In industrial settings, the production of this compound may involve large-scale nucleophilic substitution reactions using azide salts like sodium azide or potassium azide. The reaction conditions are optimized to ensure high yield and purity of the final product. The compound is then purified using techniques such as column chromatography or recrystallization .
Chemical Reactions Analysis
Types of Reactions
S-Acetyl-PEG3-azide undergoes several types of chemical reactions, including:
Click Chemistry Reactions: The azide group reacts with alkynes in the presence of a copper catalyst to form stable triazole linkages.
Reduction Reactions: The azide group can be reduced to an amine using reducing agents such as lithium aluminum hydride (LiAlH₄) or catalytic hydrogenation.
Substitution Reactions: The azide group can participate in nucleophilic substitution reactions, where it displaces other leaving groups.
Common Reagents and Conditions
Copper Catalysts: Used in click chemistry reactions to facilitate the formation of triazole linkages.
Reducing Agents: Such as lithium aluminum hydride or palladium on carbon (Pd/C) for the reduction of the azide group to an amine.
Polar Aprotic Solvents: Such as acetonitrile or dimethyl sulfoxide to enhance the nucleophilicity of the azide ion.
Major Products Formed
Triazole Linkages: Formed during click chemistry reactions.
Primary Amines: Formed during the reduction of the azide group.
Scientific Research Applications
Drug Delivery Systems
S-Acetyl-PEG3-azide is utilized in developing advanced drug delivery systems. Its ability to form stable linkages allows for the precise attachment of therapeutic agents to targeting moieties, enhancing the delivery efficacy to specific tissues or cells.
Case Study : A study demonstrated the use of this compound in constructing prodrugs that selectively target cancer cells through bioorthogonal activation. The azide-modified sialic acid derivatives were synthesized to label cancer cells selectively, leading to improved activation of phosphine-modified prodrugs .
Bioconjugation
The compound plays a crucial role in bioconjugation processes where biomolecules are modified for various applications, including imaging and therapeutic interventions.
Case Study : In a research project focusing on peptide-based therapeutics, this compound was employed to conjugate peptides with imaging agents, allowing for enhanced visualization of tumor sites in vivo .
Click Chemistry Applications
This compound is pivotal in click chemistry due to its azide group, which allows for rapid and efficient reactions with various alkyne-containing compounds.
Applications Include :
Mechanism of Action
The primary mechanism of action of S-Acetyl-PEG3-azide involves its azide group, which participates in click chemistry reactions. The azide group reacts with alkynes to form stable triazole linkages, facilitating the joining of molecules. This reaction is highly specific and efficient, making it ideal for various applications in chemistry and biology .
Comparison with Similar Compounds
Similar Compounds
Biotin-PEG4-amine: A PEG derivative with a biotin group, used for biotinylation and increasing solubility in aqueous media.
Uniqueness
S-Acetyl-PEG3-azide is unique due to its combination of an azide group and a sulfur acetyl group, which enhances its solubility and reactivity in aqueous media. This makes it particularly useful for applications requiring high solubility and efficient chemical reactions .
Biological Activity
S-Acetyl-PEG3-azide is a compound that has garnered attention for its potential applications in bioconjugation and drug delivery systems, particularly due to its bioorthogonal reactivity. This article explores the biological activity of this compound, highlighting its synthesis, mechanisms of action, and relevant case studies.
This compound is a polyethylene glycol (PEG) derivative featuring an azide functional group. The azide moiety is crucial for bioorthogonal reactions, allowing for selective labeling and conjugation in biological systems. The synthesis typically involves the acetylation of PEG followed by the introduction of the azide group, which can be achieved through various chemical reactions.
Key Characteristics:
- Molecular Formula: C₁₃H₁₉N₃O₃
- Molecular Weight: 273.31 g/mol
- CAS Number: 1310827-26-9
This compound participates in bioorthogonal reactions, most notably the Staudinger ligation and the azide-alkyne cycloaddition (click chemistry). These reactions enable the selective modification of biomolecules without interfering with native cellular processes.
- Staudinger Ligation : This reaction involves the conversion of an azide to an amine in the presence of a phosphine catalyst, facilitating the attachment of drugs or imaging agents to biomolecules.
- Click Chemistry : The azide can react with terminal alkynes to form stable triazole linkages, which are useful in various applications including drug delivery and biochemical labeling.
1. Drug Delivery Systems
This compound has been utilized in designing prodrugs that can be activated selectively in target cells. A notable study demonstrated its use in a breast cancer-targeting prodrug system where azide-modified sialic acid derivatives were employed to enhance drug delivery specifically to cancer cells.
Table 1: Prodrug Activation and Efficacy
Prodrug | IC50 (MCF-7 Cells) | IC50 (Control Cells) | Activation Mechanism |
---|---|---|---|
Doxorubicin Prodrug | 0.2 μM | 4.6 μM | Staudinger Ligation |
N-Mustard Prodrug | Complex Profile | Not Applicable | Staudinger Ligation |
The results indicated that the incorporation of azide moieties significantly increased the selectivity and efficacy of drug activation in cancer cells compared to normal cells .
2. Metabolic Labeling
The azide functionality allows for metabolic labeling of sialylated glycoconjugates on cell surfaces. This technique aids in studying cell interactions and dynamics within their microenvironment. A comparative study highlighted how switching between azide and alkyne tags can enhance detection methods for metabolites and post-translational modifications .
Case Studies
Case Study 1: Targeted Cancer Therapy
In a recent study, researchers utilized this compound to develop a targeted therapy for breast cancer by attaching cytotoxic agents specifically to cancer cells via bioorthogonal chemistry. The study demonstrated successful selective activation of prodrugs using the Staudinger reaction, showcasing the potential for reduced side effects compared to traditional chemotherapeutics .
Case Study 2: Glycoconjugate Labeling
Another significant application involved using this compound for labeling sialic acid residues on glycoproteins. This method facilitated enhanced visualization of glycan structures on cell surfaces, providing insights into cellular communication mechanisms and potential therapeutic targets .
Q & A
Basic Research Questions
Q. What is the primary synthetic route for S-Acetyl-PEG3-azide, and how does its structure influence reactivity in bioconjugation?
this compound is synthesized via functionalization of polyethylene glycol (PEG) with an azide group and an S-acetyl-protected thiol. The PEG spacer enhances solubility in polar solvents (e.g., water, DMSO), while the azide enables copper-catalyzed azide-alkyne cycloaddition (CuAAC) for biomolecule labeling . The S-acetyl group acts as a protecting moiety, preventing premature thiol oxidation or disulfide bond formation. Structural analysis via NMR and mass spectrometry is critical to confirm purity and functional group integrity .
Q. How can researchers experimentally validate the solubility and stability of this compound in aqueous systems?
Solubility is assessed by dissolving the compound in water or buffered solutions (e.g., PBS) at varying concentrations, followed by dynamic light scattering (DLS) or turbidity measurements. Stability studies involve monitoring azide group integrity (via FTIR or Raman spectroscopy) and thiol deprotection kinetics (using Ellman’s assay) under physiological conditions (pH 7.4, 37°C) .
Q. What are the standard protocols for removing the S-acetyl protecting group prior to conjugation?
The S-acetyl group is cleaved under mild basic conditions (e.g., 0.1 M hydroxylamine, pH 7–8, 30 minutes at 25°C). Reaction progress is tracked via HPLC or thiol-specific fluorogenic probes. Post-deprotection, the free thiol is stabilized with EDTA to prevent metal-catalyzed oxidation .
Advanced Research Questions
Q. How do structural variations in PEG-azide analogs (e.g., PEG length, branching) impact CuAAC efficiency and biomolecule labeling specificity?
Longer PEG chains (e.g., PEG11 vs. PEG3) reduce steric hindrance in bulky targets but may decrease reaction rates due to lower azide local concentration. Branching introduces multivalency, enhancing binding avidity but complicating purification. Kinetic studies using stopped-flow spectroscopy and competitive binding assays are recommended to quantify trade-offs .
Q. What experimental strategies resolve contradictions in reported CuAAC yields when using this compound with heterogeneous biomolecules?
Discrepancies often arise from variable copper catalyst purity, competing side reactions (e.g., thiol-azide interactions), or incomplete deprotection. Systematic optimization includes:
- Testing copper(I) sources (e.g., TBTA vs. THPTA ligands) to minimize cytotoxicity .
- Pre-incubating biomolecules with EDTA to chelate residual metals .
- Validating deprotection efficiency via tandem LC-MS and kinetic modeling .
Q. How can researchers design a controlled experiment to compare this compound with alternative click chemistry reagents (e.g., DBCO-PEG3-azide) in live-cell labeling?
- Hypothesis : DBCO’s copper-free reactivity improves cell viability but sacrifices reaction speed.
- Method : Parallel labeling of cell-surface proteins using equimolar reagent concentrations. Monitor kinetics via time-lapse fluorescence microscopy and viability via ATP assays.
- Analysis : Quantify labeling efficiency (flow cytometry) and nonspecific binding (negative controls with azide-free cells) .
Q. What analytical techniques are most reliable for confirming successful conjugation of this compound to peptide backbones?
- Primary validation : MALDI-TOF MS to detect mass shifts corresponding to PEG3-azide addition.
- Secondary validation : X-ray crystallography or cryo-EM for structural confirmation in complex systems.
- Tertiary validation : Functional assays (e.g., binding affinity via SPR) to ensure retained bioactivity post-conjugation .
Q. Methodological Best Practices
-
Data Presentation : Use tables to compare reaction parameters (e.g., pH, temperature) and outcomes (yield, purity). For example:
Condition Yield (%) Purity (%) Side Products Identified CuSO4/THPTA 92 98 None CuBr/TBTA 85 95 Oxidized thiols -
Critical Analysis : Address limitations (e.g., copper toxicity in vivo) and propose alternatives (e.g., strain-promoted azide-alkyne cycloaddition) .
-
Reproducibility : Document batch-specific variations (e.g., azide content via titration) and share raw data in supplementary materials .
Properties
IUPAC Name |
S-[2-[2-[2-(2-azidoethoxy)ethoxy]ethoxy]ethyl] ethanethioate | |
---|---|---|
Source | PubChem | |
URL | https://pubchem.ncbi.nlm.nih.gov | |
Description | Data deposited in or computed by PubChem | |
InChI |
InChI=1S/C10H19N3O4S/c1-10(14)18-9-8-17-7-6-16-5-4-15-3-2-12-13-11/h2-9H2,1H3 | |
Source | PubChem | |
URL | https://pubchem.ncbi.nlm.nih.gov | |
Description | Data deposited in or computed by PubChem | |
InChI Key |
PIXCCFAIMVRUDJ-UHFFFAOYSA-N | |
Source | PubChem | |
URL | https://pubchem.ncbi.nlm.nih.gov | |
Description | Data deposited in or computed by PubChem | |
Canonical SMILES |
CC(=O)SCCOCCOCCOCCN=[N+]=[N-] | |
Source | PubChem | |
URL | https://pubchem.ncbi.nlm.nih.gov | |
Description | Data deposited in or computed by PubChem | |
Molecular Formula |
C10H19N3O4S | |
Source | PubChem | |
URL | https://pubchem.ncbi.nlm.nih.gov | |
Description | Data deposited in or computed by PubChem | |
Molecular Weight |
277.34 g/mol | |
Source | PubChem | |
URL | https://pubchem.ncbi.nlm.nih.gov | |
Description | Data deposited in or computed by PubChem | |
Retrosynthesis Analysis
AI-Powered Synthesis Planning: Our tool employs the Template_relevance Pistachio, Template_relevance Bkms_metabolic, Template_relevance Pistachio_ringbreaker, Template_relevance Reaxys, Template_relevance Reaxys_biocatalysis model, leveraging a vast database of chemical reactions to predict feasible synthetic routes.
One-Step Synthesis Focus: Specifically designed for one-step synthesis, it provides concise and direct routes for your target compounds, streamlining the synthesis process.
Accurate Predictions: Utilizing the extensive PISTACHIO, BKMS_METABOLIC, PISTACHIO_RINGBREAKER, REAXYS, REAXYS_BIOCATALYSIS database, our tool offers high-accuracy predictions, reflecting the latest in chemical research and data.
Strategy Settings
Precursor scoring | Relevance Heuristic |
---|---|
Min. plausibility | 0.01 |
Model | Template_relevance |
Template Set | Pistachio/Bkms_metabolic/Pistachio_ringbreaker/Reaxys/Reaxys_biocatalysis |
Top-N result to add to graph | 6 |
Feasible Synthetic Routes
Disclaimer and Information on In-Vitro Research Products
Please be aware that all articles and product information presented on BenchChem are intended solely for informational purposes. The products available for purchase on BenchChem are specifically designed for in-vitro studies, which are conducted outside of living organisms. In-vitro studies, derived from the Latin term "in glass," involve experiments performed in controlled laboratory settings using cells or tissues. It is important to note that these products are not categorized as medicines or drugs, and they have not received approval from the FDA for the prevention, treatment, or cure of any medical condition, ailment, or disease. We must emphasize that any form of bodily introduction of these products into humans or animals is strictly prohibited by law. It is essential to adhere to these guidelines to ensure compliance with legal and ethical standards in research and experimentation.