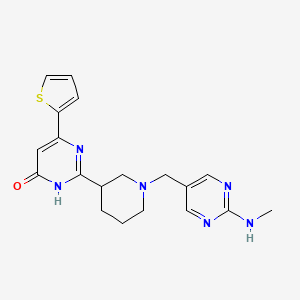
Ribocil
- Click on QUICK INQUIRY to receive a quote from our team of experts.
- With the quality product at a COMPETITIVE price, you can focus more on your research.
Overview
Description
Ribocil is a synthetic small molecule that functions as a highly selective chemical modulator of bacterial riboflavin riboswitches. It was identified through a phenotypic screen targeting the riboflavin biosynthesis pathway in Gram-negative bacteria, specifically Escherichia coli. This compound mimics the natural ligand flavin mononucleotide (FMN) and inhibits riboswitch-mediated gene expression, thereby repressing bacterial growth .
Mechanism of Action
Target of Action
Ribocil primarily targets the FMN riboswitch . Riboswitches are non-coding RNA structural elements that direct gene expression in numerous metabolic pathways . The FMN riboswitch is a regulatory RNA structural element that specifically binds natural ligands to regulate gene expression .
Mode of Action
This compound functions as a potent and highly selective synthetic mimic of the natural ligand, FMN, to repress riboswitch-mediated gene expression . It interacts with the riboswitch through key face-to-face stacking interactions between the 6-thiophenyl-pyrimidonyl group of this compound and nucleotides A48 and A85, and a hydrogen bond interaction between the pyrimidonal oxygen of this compound and nucleotides A48 and A99 .
Biochemical Pathways
This compound affects the riboflavin biosynthesis pathway . Riboflavin (vitamin B2) concentrations in bacteria are regulated by FMN riboswitches, which control the expression of genes required for the biosynthesis and transport of this essential vitamin . This compound, by mimicking FMN, inhibits this pathway, thereby affecting the production of riboflavin .
Pharmacokinetics
It’s important to note that these properties play a crucial role in the bioavailability of a drug .
Result of Action
This compound’s action results in the repression of riboswitch-mediated gene expression and inhibition of bacterial growth . By mimicking FMN and binding to the FMN riboswitch, this compound inhibits the riboflavin biosynthesis pathway, thereby affecting the production of riboflavin and inhibiting bacterial growth .
Biochemical Analysis
Biochemical Properties
Ribocil functions by mimicking the natural ligand, flavin mononucleotide, and binding to the FMN riboswitch. This interaction inhibits the riboswitch-mediated gene expression essential for riboflavin biosynthesis. This compound specifically interacts with the FMN riboswitch aptamer domain, inducing a conformational change that represses the expression of genes involved in riboflavin biosynthesis . The primary biomolecules this compound interacts with include the FMN riboswitch RNA and the ribB gene product .
Cellular Effects
This compound exerts its effects on bacterial cells by inhibiting the biosynthesis of riboflavin, an essential vitamin for bacterial growth and metabolism. This inhibition leads to a decrease in the levels of riboflavin, flavin mononucleotide, and flavin adenine dinucleotide within the cell . Consequently, this compound disrupts cellular processes dependent on these cofactors, including cellular metabolism and gene expression .
Molecular Mechanism
At the molecular level, this compound binds to the FMN riboswitch with high specificity and affinity. This binding induces a conformational change in the riboswitch, preventing the transcription of downstream genes involved in riboflavin biosynthesis . This compound’s mechanism of action involves competitive inhibition, where it competes with flavin mononucleotide for binding to the riboswitch .
Temporal Effects in Laboratory Settings
In laboratory settings, this compound has been shown to maintain its inhibitory effects over time. Studies have demonstrated that this compound remains stable and effective in inhibiting bacterial growth over extended periods . Resistance can develop through mutations in the FMN riboswitch, which may reduce this compound’s binding affinity .
Dosage Effects in Animal Models
In animal models, the effects of this compound vary with dosage. At lower doses, this compound effectively inhibits bacterial growth without significant toxicity . At higher doses, adverse effects such as toxicity and disruption of normal cellular functions have been observed . The therapeutic window for this compound is thus critical for its effective use as an antibacterial agent.
Metabolic Pathways
This compound is involved in the metabolic pathway of riboflavin biosynthesis. By inhibiting the FMN riboswitch, this compound disrupts the production of riboflavin and its derivatives, flavin mononucleotide and flavin adenine dinucleotide . This inhibition affects the overall metabolic flux and reduces the availability of these essential cofactors for various enzymatic reactions .
Transport and Distribution
Within bacterial cells, this compound is transported and distributed primarily through passive diffusion. Once inside the cell, this compound localizes to the cytoplasm, where it interacts with the FMN riboswitch . The distribution of this compound within the cell is influenced by its chemical properties and the presence of specific transporters or binding proteins .
Subcellular Localization
This compound predominantly localizes in the cytoplasm of bacterial cells, where it exerts its inhibitory effects on the FMN riboswitch . The subcellular localization of this compound is crucial for its function, as it needs to be in proximity to the riboswitch to effectively inhibit riboflavin biosynthesis .
Preparation Methods
Synthetic Routes and Reaction Conditions: Ribocil is synthesized through a series of chemical reactions involving the formation of its core structure and subsequent functionalization. The synthetic route typically involves the use of various organic reagents and catalysts under controlled conditions to achieve the desired product. Specific details on the synthetic route and reaction conditions are proprietary and not publicly disclosed .
Industrial Production Methods: Industrial production of this compound involves scaling up the laboratory synthesis to a larger scale, ensuring consistency and purity of the final product. This process includes optimization of reaction conditions, purification steps, and quality control measures to meet industrial standards .
Chemical Reactions Analysis
Types of Reactions: Ribocil undergoes several types of chemical reactions, including:
Oxidation: this compound can be oxidized under specific conditions to form oxidized derivatives.
Reduction: Reduction reactions can modify this compound’s functional groups, potentially altering its biological activity.
Substitution: Substitution reactions can introduce different functional groups into this compound’s structure, leading to the formation of analogs with varying properties
Common Reagents and Conditions: Common reagents used in these reactions include oxidizing agents (e.g., hydrogen peroxide), reducing agents (e.g., sodium borohydride), and various organic solvents. Reaction conditions such as temperature, pH, and reaction time are carefully controlled to achieve the desired outcomes .
Major Products: The major products formed from these reactions include various analogs of this compound with modified functional groups. These analogs are often studied for their potential biological activities and therapeutic applications .
Scientific Research Applications
Ribocil has a wide range of scientific research applications, including:
Chemistry: this compound is used as a tool compound to study riboswitch-mediated gene regulation and to develop new chemical probes targeting RNA structures
Biology: In biological research, this compound is used to investigate the role of riboswitches in bacterial metabolism and gene expression
Medicine: this compound’s ability to inhibit bacterial growth makes it a potential candidate for the development of new antibiotics, particularly against multi-drug resistant bacteria
Industry: this compound is used in the development of new antibacterial agents and in the study of bacterial resistance mechanisms
Comparison with Similar Compounds
Flavin Mononucleotide (FMN): The natural ligand for the FMN riboswitch, structurally similar to Ribocil but with different binding properties
This compound-B: An analog of this compound with superior microbiological activity and binding affinity to the FMN riboswitch
This compound-C: Another analog with distinct chemical properties and biological activities.
Uniqueness of this compound: this compound is unique in its ability to selectively mimic the natural ligand FMN and inhibit riboswitch-mediated gene expression. Its structural distinctiveness from FMN and its potent antibacterial activity make it a valuable tool in the study of riboswitches and the development of new antibiotics .
Properties
IUPAC Name |
2-[1-[[2-(methylamino)pyrimidin-5-yl]methyl]piperidin-3-yl]-4-thiophen-2-yl-1H-pyrimidin-6-one |
Source
|
---|---|---|
Source | PubChem | |
URL | https://pubchem.ncbi.nlm.nih.gov | |
Description | Data deposited in or computed by PubChem | |
InChI |
InChI=1S/C19H22N6OS/c1-20-19-21-9-13(10-22-19)11-25-6-2-4-14(12-25)18-23-15(8-17(26)24-18)16-5-3-7-27-16/h3,5,7-10,14H,2,4,6,11-12H2,1H3,(H,20,21,22)(H,23,24,26) |
Source
|
Source | PubChem | |
URL | https://pubchem.ncbi.nlm.nih.gov | |
Description | Data deposited in or computed by PubChem | |
InChI Key |
ZSXCVAIJFUEGJR-UHFFFAOYSA-N |
Source
|
Source | PubChem | |
URL | https://pubchem.ncbi.nlm.nih.gov | |
Description | Data deposited in or computed by PubChem | |
Canonical SMILES |
CNC1=NC=C(C=N1)CN2CCCC(C2)C3=NC(=CC(=O)N3)C4=CC=CS4 |
Source
|
Source | PubChem | |
URL | https://pubchem.ncbi.nlm.nih.gov | |
Description | Data deposited in or computed by PubChem | |
Molecular Formula |
C19H22N6OS |
Source
|
Source | PubChem | |
URL | https://pubchem.ncbi.nlm.nih.gov | |
Description | Data deposited in or computed by PubChem | |
Molecular Weight |
382.5 g/mol |
Source
|
Source | PubChem | |
URL | https://pubchem.ncbi.nlm.nih.gov | |
Description | Data deposited in or computed by PubChem | |
Disclaimer and Information on In-Vitro Research Products
Please be aware that all articles and product information presented on BenchChem are intended solely for informational purposes. The products available for purchase on BenchChem are specifically designed for in-vitro studies, which are conducted outside of living organisms. In-vitro studies, derived from the Latin term "in glass," involve experiments performed in controlled laboratory settings using cells or tissues. It is important to note that these products are not categorized as medicines or drugs, and they have not received approval from the FDA for the prevention, treatment, or cure of any medical condition, ailment, or disease. We must emphasize that any form of bodily introduction of these products into humans or animals is strictly prohibited by law. It is essential to adhere to these guidelines to ensure compliance with legal and ethical standards in research and experimentation.