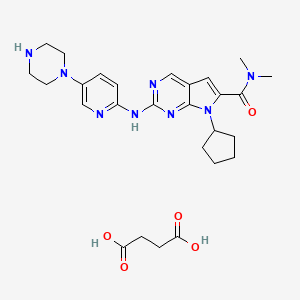
Ribociclib succinate
Overview
Description
Ribociclib succinate is a selective cyclin-dependent kinase inhibitor, specifically targeting cyclin-dependent kinase 4 and cyclin-dependent kinase 6. These proteins play a crucial role in cell cycle regulation, and their over-activation can lead to uncontrolled cell proliferation, a hallmark of cancer. This compound is primarily used in the treatment of hormone receptor-positive, human epidermal growth factor receptor 2-negative advanced or metastatic breast cancer .
Mechanism of Action
Target of Action
Ribociclib succinate is a selective cyclin-dependent kinase inhibitor . It primarily targets two proteins, cyclin-dependent kinase 4 and 6 (CDK4/6) . These proteins play a crucial role in cell cycle regulation and, when over-activated, can enable cancer cells to grow and divide too quickly .
Mode of Action
This compound interacts with its targets (CDK4/6) by inhibiting their activity . This inhibition slows down the progression of cancer by preventing the over-activation of CDK4/6 .
Biochemical Pathways
The primary biochemical pathway affected by this compound is the cyclin D–CDK4/6–p16–retinoblastoma (Rb) pathway . This pathway is commonly disrupted in cancer, leading to abnormal cell proliferation . In this pathway, cyclin D is the key entry point at which various mitogenic and growth arrest signaling pathways converge to regulate the cell cycle . In response to mitogenic signaling, levels of D-type cyclins rise and associate with CDK4 or CDK6 .
Pharmacokinetics
This compound is orally bioavailable . Following oral dosing, ribociclib is rapidly absorbed with median Tmax ranging from 1 to 5 hours . It is extensively metabolized by cytochrome P450 3A4 . The exposure of this compound is dramatically affected by strong cytochrome P450 3A4 modulators .
Result of Action
The molecular and cellular effects of this compound’s action primarily involve the inhibition of cell proliferation. By inhibiting CDK4/6, this compound prevents the over-activation of these proteins, thereby slowing down the growth and division of cancer cells . This compound has been shown to induce cytostasis at nanomolar concentrations in certain cancer cell lines, suggesting that these cells might be particularly susceptible to CDK4/6 inhibition .
Action Environment
The action, efficacy, and stability of this compound can be influenced by various environmental factors. For instance, the presence of strong cytochrome P450 3A4 modulators can dramatically affect the exposure of this compound . Additionally, the susceptibility of certain cancer cells to CDK4/6 inhibition may vary depending on the specific cellular environment .
Biochemical Analysis
Biochemical Properties
Ribociclib succinate plays a crucial role in biochemical reactions by inhibiting the activity of cyclin-dependent kinase 4 and 6 (CDK4/6). These enzymes are essential for cell cycle progression, particularly the transition from the G1 phase to the S phase. By binding to CDK4/6, this compound prevents their interaction with cyclin D, thereby inhibiting the phosphorylation of the retinoblastoma protein (Rb). This inhibition leads to cell cycle arrest in the G1 phase, preventing cancer cells from proliferating .
Cellular Effects
This compound exerts significant effects on various types of cells and cellular processes. It primarily influences cell function by inducing cell cycle arrest at the G1 phase. This action is achieved through the inhibition of CDK4/6, which prevents the phosphorylation of the retinoblastoma protein (Rb). As a result, the cell cycle is halted, and cancer cells are unable to proliferate. Additionally, this compound has been shown to impact cell signaling pathways, gene expression, and cellular metabolism, further contributing to its antitumor effects .
Molecular Mechanism
The molecular mechanism of this compound involves its selective inhibition of CDK4/6. By binding to these kinases, this compound prevents their interaction with cyclin D, thereby inhibiting the phosphorylation of the retinoblastoma protein (Rb). This inhibition leads to cell cycle arrest in the G1 phase, preventing cancer cells from proliferating. Additionally, this compound has been shown to induce apoptosis and senescence in cancer cells, further contributing to its antitumor effects .
Dosage Effects in Animal Models
The effects of this compound vary with different dosages in animal models. At lower doses, this compound effectively inhibits CDK4/6 activity, leading to cell cycle arrest and tumor growth suppression. At higher doses, this compound may cause toxic or adverse effects, such as neutropenia and corrected QT prolongation. These threshold effects highlight the importance of dose optimization to maximize therapeutic efficacy while minimizing toxicity .
Metabolic Pathways
This compound is extensively metabolized by cytochrome P450 3A4 (CYP3A4) in the liver. The primary metabolic pathways involve hydroxylation, oxidation, and reduction, leading to the formation of various metabolites. These metabolites are further processed and eliminated from the body. The involvement of CYP3A4 in the metabolism of this compound underscores the potential for drug-drug interactions, particularly with strong CYP3A4 modulators .
Transport and Distribution
This compound is transported and distributed within cells and tissues through various mechanisms. It is a substrate for the efflux transporter P-glycoprotein (P-gp), which limits its penetration into the brain. This efflux transporter plays a crucial role in the pharmacokinetics of this compound, affecting its distribution and accumulation in different tissues. Understanding these transport mechanisms is essential for optimizing the therapeutic efficacy of this compound .
Subcellular Localization
The subcellular localization of this compound is primarily within the cytoplasm, where it interacts with CDK4/6 and inhibits their activity. This localization is crucial for its function, as it allows this compound to effectively inhibit the phosphorylation of the retinoblastoma protein (Rb) and induce cell cycle arrest. Additionally, this compound may undergo post-translational modifications that influence its activity and localization within specific cellular compartments .
Preparation Methods
Synthetic Routes and Reaction Conditions
Ribociclib succinate is synthesized through a multi-step chemical process. The synthesis involves the formation of the core pyrrolo[2,3-d]pyrimidine structure, followed by the introduction of various functional groups. Key steps include:
Formation of the Pyrrolo[2,3-d]pyrimidine Core: This involves the cyclization of appropriate precursors under controlled conditions.
Functional Group Introduction: Various functional groups, such as the cyclopentyl and piperazine moieties, are introduced through nucleophilic substitution and other reactions.
Final Coupling and Purification: The final product is obtained through coupling reactions, followed by purification using techniques like high-performance liquid chromatography.
Industrial Production Methods
Industrial production of this compound involves scaling up the laboratory synthesis process. This includes optimizing reaction conditions to ensure high yield and purity, as well as implementing stringent quality control measures. The use of eco-friendly solvents and green chemistry principles is also explored to minimize environmental impact .
Chemical Reactions Analysis
Types of Reactions
Ribociclib succinate undergoes various chemical reactions, including:
Oxidation: this compound can be oxidized to form hydroxylated metabolites.
Reduction: Reduction reactions can lead to the formation of reduced metabolites.
Substitution: Nucleophilic substitution reactions are used in the synthesis of this compound.
Common Reagents and Conditions
Oxidation: Common oxidizing agents include hydrogen peroxide and cytochrome P450 enzymes.
Reduction: Reducing agents such as sodium borohydride are used.
Substitution: Nucleophiles like amines and alcohols are employed under controlled conditions.
Major Products Formed
The major products formed from these reactions include various hydroxylated and reduced metabolites, which are further characterized using techniques like mass spectrometry .
Scientific Research Applications
Ribociclib succinate has a wide range of scientific research applications:
Chemistry: Used as a model compound to study cyclin-dependent kinase inhibition and its effects on cell cycle regulation.
Biology: Investigated for its role in modulating cell proliferation and apoptosis in various cell lines.
Medicine: Primarily used in the treatment of advanced breast cancer. Ongoing research explores its potential in other cancer types.
Industry: Utilized in the development of targeted cancer therapies and combination treatments
Comparison with Similar Compounds
Ribociclib succinate is part of a class of drugs known as cyclin-dependent kinase inhibitors. Similar compounds include:
Palbociclib: Another cyclin-dependent kinase 4 and cyclin-dependent kinase 6 inhibitor used in the treatment of breast cancer.
Abemaciclib: A cyclin-dependent kinase 4 and cyclin-dependent kinase 6 inhibitor with a slightly different pharmacokinetic profile and additional activity against other kinases.
Uniqueness
This compound is unique due to its high selectivity for cyclin-dependent kinase 4 and cyclin-dependent kinase 6, which results in a favorable safety and efficacy profile. It is often used in combination with other targeted therapies to enhance clinical outcomes and delay the development of treatment resistance .
Properties
IUPAC Name |
butanedioic acid;7-cyclopentyl-N,N-dimethyl-2-[(5-piperazin-1-ylpyridin-2-yl)amino]pyrrolo[2,3-d]pyrimidine-6-carboxamide | |
---|---|---|
Source | PubChem | |
URL | https://pubchem.ncbi.nlm.nih.gov | |
Description | Data deposited in or computed by PubChem | |
InChI |
InChI=1S/C23H30N8O.C4H6O4/c1-29(2)22(32)19-13-16-14-26-23(28-21(16)31(19)17-5-3-4-6-17)27-20-8-7-18(15-25-20)30-11-9-24-10-12-30;5-3(6)1-2-4(7)8/h7-8,13-15,17,24H,3-6,9-12H2,1-2H3,(H,25,26,27,28);1-2H2,(H,5,6)(H,7,8) | |
Source | PubChem | |
URL | https://pubchem.ncbi.nlm.nih.gov | |
Description | Data deposited in or computed by PubChem | |
InChI Key |
NHANOMFABJQAAH-UHFFFAOYSA-N | |
Source | PubChem | |
URL | https://pubchem.ncbi.nlm.nih.gov | |
Description | Data deposited in or computed by PubChem | |
Canonical SMILES |
CN(C)C(=O)C1=CC2=CN=C(N=C2N1C3CCCC3)NC4=NC=C(C=C4)N5CCNCC5.C(CC(=O)O)C(=O)O | |
Source | PubChem | |
URL | https://pubchem.ncbi.nlm.nih.gov | |
Description | Data deposited in or computed by PubChem | |
Molecular Formula |
C27H36N8O5 | |
Source | PubChem | |
URL | https://pubchem.ncbi.nlm.nih.gov | |
Description | Data deposited in or computed by PubChem | |
DSSTOX Substance ID |
DTXSID301027923 | |
Record name | Ribociclib succinate | |
Source | EPA DSSTox | |
URL | https://comptox.epa.gov/dashboard/DTXSID301027923 | |
Description | DSSTox provides a high quality public chemistry resource for supporting improved predictive toxicology. | |
Molecular Weight |
552.6 g/mol | |
Source | PubChem | |
URL | https://pubchem.ncbi.nlm.nih.gov | |
Description | Data deposited in or computed by PubChem | |
CAS No. |
1374639-75-4 | |
Record name | Ribociclib succinate [USAN] | |
Source | ChemIDplus | |
URL | https://pubchem.ncbi.nlm.nih.gov/substance/?source=chemidplus&sourceid=1374639754 | |
Description | ChemIDplus is a free, web search system that provides access to the structure and nomenclature authority files used for the identification of chemical substances cited in National Library of Medicine (NLM) databases, including the TOXNET system. | |
Record name | Ribociclib succinate | |
Source | EPA DSSTox | |
URL | https://comptox.epa.gov/dashboard/DTXSID301027923 | |
Description | DSSTox provides a high quality public chemistry resource for supporting improved predictive toxicology. | |
Record name | 7-cyclopentyl-N,N-dimethyl-2-{[5-(piperazin-1-yl)pyridin-2-yl]amino}-7H-pyrrolo[2,3-d]pyrimidine-6-carboxamide butanedioate | |
Source | European Chemicals Agency (ECHA) | |
URL | https://echa.europa.eu/information-on-chemicals | |
Description | The European Chemicals Agency (ECHA) is an agency of the European Union which is the driving force among regulatory authorities in implementing the EU's groundbreaking chemicals legislation for the benefit of human health and the environment as well as for innovation and competitiveness. | |
Explanation | Use of the information, documents and data from the ECHA website is subject to the terms and conditions of this Legal Notice, and subject to other binding limitations provided for under applicable law, the information, documents and data made available on the ECHA website may be reproduced, distributed and/or used, totally or in part, for non-commercial purposes provided that ECHA is acknowledged as the source: "Source: European Chemicals Agency, http://echa.europa.eu/". Such acknowledgement must be included in each copy of the material. ECHA permits and encourages organisations and individuals to create links to the ECHA website under the following cumulative conditions: Links can only be made to webpages that provide a link to the Legal Notice page. | |
Record name | RIBOCICLIB SUCCINATE | |
Source | FDA Global Substance Registration System (GSRS) | |
URL | https://gsrs.ncats.nih.gov/ginas/app/beta/substances/BG7HLX2919 | |
Description | The FDA Global Substance Registration System (GSRS) enables the efficient and accurate exchange of information on what substances are in regulated products. Instead of relying on names, which vary across regulatory domains, countries, and regions, the GSRS knowledge base makes it possible for substances to be defined by standardized, scientific descriptions. | |
Explanation | Unless otherwise noted, the contents of the FDA website (www.fda.gov), both text and graphics, are not copyrighted. They are in the public domain and may be republished, reprinted and otherwise used freely by anyone without the need to obtain permission from FDA. Credit to the U.S. Food and Drug Administration as the source is appreciated but not required. | |
Q1: What analytical techniques were employed in the study to investigate the pH-dependent interaction between ribociclib succinate and acid-reducing agents?
A: The researchers developed a robust and accurate analytical method using a three-level three-factorial box-behnken design to quantify this compound in micro-dissolution samples. This approach, following the Analytical Quality by Design (AQbD) principles, ensured the reliability and accuracy of the solubility measurements across the different pH conditions tested. The study identified the pH of the aqueous mobile phase and flow rate as critical process parameters influencing the method's performance. []
Disclaimer and Information on In-Vitro Research Products
Please be aware that all articles and product information presented on BenchChem are intended solely for informational purposes. The products available for purchase on BenchChem are specifically designed for in-vitro studies, which are conducted outside of living organisms. In-vitro studies, derived from the Latin term "in glass," involve experiments performed in controlled laboratory settings using cells or tissues. It is important to note that these products are not categorized as medicines or drugs, and they have not received approval from the FDA for the prevention, treatment, or cure of any medical condition, ailment, or disease. We must emphasize that any form of bodily introduction of these products into humans or animals is strictly prohibited by law. It is essential to adhere to these guidelines to ensure compliance with legal and ethical standards in research and experimentation.