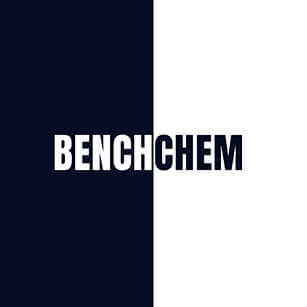
Rho-IA
Overview
Description
Rho-IA, also known as iodoacetamide rhodamine, is a fluorescent probe widely used in biochemical and molecular biology research. It is particularly valuable for labeling cysteine residues in proteins, allowing for the visualization and study of protein structures and functions.
Mechanism of Action
Target of Action
Rho-IA, also known as Rho(D) immune globulin, is a solution of antibodies used to prevent isoimmunization of Rho(D) negative patients exposed to Rho(D) positive blood in pregnancy or transfusion . The primary targets of this compound are the Rho family proteins, which are crucial for several reasons: they control almost all fundamental cellular processes in eukaryotes including morphogenesis, polarity, movement, cell division, gene expression, and cytoskeleton reorganization .
Mode of Action
This compound acts as a molecular switch that is activated in response to binding of chemokines, cytokines, and growth factors . It interacts with its targets and causes changes in the cell. For instance, it has been shown to increase platelet counts and reduce bleeding in Rho-positive patients with immune thrombocytopenic purpura (ITP) by inhibiting autoantibody-mediated platelet clearance .
Biochemical Pathways
This compound affects the RHO GTPase functions and many studies have convincingly demonstrated that altered signal transduction through RHO GTPases is a recurring theme in the progression of human malignancies . It seems that 20 canonical RHO GTPases are likely regulated by three GDIs, 85 GEFs, and 66 GAPs, and eventually interact with > downstream effectors .
Pharmacokinetics
Monoclonal antibodies like this compound typically have a complex pharmacokinetic profile influenced by factors such as the route of administration, the dose, and the patient’s immune response . They are usually administered intravenously and have a relatively long half-life, allowing for less frequent dosing .
Result of Action
The molecular and cellular effects of this compound’s action are diverse. It has been shown to promote neoplastic transformation and cell proliferation, whereas its deficiency inhibited development . In the context of immune response, this compound plays a key role in immune cell differentiation and function .
Action Environment
Environmental factors can influence the action, efficacy, and stability of this compound. For instance, it has been suggested that controlling the action of the prokaryotic Rho factor could generate major biotechnological improvements, such as an increase in bacterial productivity or a reduction of the microbial-specific growth rate . Additionally, the action of this compound can be influenced by the presence of other molecules in the environment, such as chemokines, cytokines, and growth factors .
Biochemical Analysis
Biochemical Properties
Rho-IA is a key regulator in biochemical reactions, particularly those involving the cytoskeleton. It interacts with several enzymes, proteins, and other biomolecules. One of the primary interactions is with Rho-associated coiled-coil containing protein kinase, which is activated by this compound and subsequently phosphorylates downstream targets. This phosphorylation event leads to the activation of cytoskeletal proteins such as myosin light chain and LIM kinase. Additionally, this compound interacts with guanine nucleotide exchange factors and GTPase-activating proteins, which regulate its activity by promoting the exchange of guanosine diphosphate for guanosine triphosphate and hydrolyzing guanosine triphosphate, respectively .
Cellular Effects
This compound exerts significant effects on various cell types and cellular processes. In fibroblasts, this compound activation leads to the formation of stress fibers and focal adhesions, which are essential for cell migration and adhesion. In neurons, this compound regulates axon guidance and dendritic spine morphology, impacting neuronal connectivity and plasticity. Furthermore, this compound influences cell signaling pathways, including the mitogen-activated protein kinase pathway, which affects gene expression and cellular metabolism. The activation of this compound in immune cells, such as macrophages and dendritic cells, enhances their ability to migrate to sites of infection and inflammation .
Molecular Mechanism
The molecular mechanism of this compound involves its activation by binding to guanosine triphosphate, which induces a conformational change that allows it to interact with downstream effectors. One of the primary effectors is Rho-associated coiled-coil containing protein kinase, which phosphorylates various substrates, leading to changes in cytoskeletal dynamics and cell motility. This compound also interacts with other signaling molecules, such as phosphatidylinositol 4,5-bisphosphate, which modulates its activity. Additionally, this compound can inhibit or activate specific enzymes, such as phosphatase and tensin homolog, affecting gene expression and cellular functions .
Temporal Effects in Laboratory Settings
In laboratory settings, the effects of this compound can change over time. This compound is relatively stable under physiological conditions, but its activity can be modulated by post-translational modifications, such as phosphorylation and ubiquitination. These modifications can lead to changes in this compound stability and degradation. Long-term studies have shown that sustained activation of this compound can result in altered cellular functions, such as increased cell migration and invasion in cancer cells. Additionally, the temporal effects of this compound can be influenced by the presence of other signaling molecules and environmental factors .
Dosage Effects in Animal Models
The effects of this compound vary with different dosages in animal models. At low doses, this compound activation can promote cell migration and wound healing. At high doses, this compound activation can lead to adverse effects, such as increased cell proliferation and tumorigenesis. Studies in animal models have shown that this compound inhibitors can reduce tumor growth and metastasis, highlighting the importance of dosage in therapeutic applications. Additionally, threshold effects have been observed, where a minimum level of this compound activation is required to elicit specific cellular responses .
Metabolic Pathways
This compound is involved in several metabolic pathways, including those regulating cytoskeletal dynamics and cell motility. It interacts with enzymes such as Rho-associated coiled-coil containing protein kinase and LIM kinase, which phosphorylate substrates involved in actin polymerization and myosin contraction. This compound also affects metabolic flux by modulating the activity of enzymes involved in glycolysis and oxidative phosphorylation. These interactions can lead to changes in metabolite levels and cellular energy production .
Transport and Distribution
This compound is transported and distributed within cells and tissues through interactions with transporters and binding proteins. It is primarily localized in the cytoplasm but can translocate to the plasma membrane upon activation. This compound interacts with guanine nucleotide exchange factors and GTPase-activating proteins, which regulate its localization and activity. Additionally, this compound can be transported to specific cellular compartments, such as the nucleus, where it can influence gene expression and cellular functions .
Subcellular Localization
The subcellular localization of this compound is critical for its activity and function. This compound is primarily localized in the cytoplasm but can translocate to the plasma membrane, nucleus, and other cellular compartments upon activation. This localization is regulated by targeting signals and post-translational modifications, such as phosphorylation and prenylation. The subcellular localization of this compound can affect its interactions with other biomolecules and its ability to regulate cellular processes .
Preparation Methods
Synthetic Routes and Reaction Conditions: The synthesis of Rho-IA involves the reaction of rhodamine with iodoacetamide. The process typically requires the following steps:
Preparation of Rhodamine: Rhodamine is synthesized through the condensation of phthalic anhydride with resorcinol, followed by the reaction with ethylamine.
Iodoacetamide Reaction: Rhodamine is then reacted with iodoacetamide in the presence of a base, such as sodium hydroxide, to form the final product, this compound.
Industrial Production Methods: Industrial production of this compound follows similar synthetic routes but on a larger scale. The process involves stringent quality control measures to ensure the purity and consistency of the compound.
Chemical Reactions Analysis
Types of Reactions: Rho-IA primarily undergoes nucleophilic substitution reactions due to the presence of the iodoacetamide group. This group reacts with thiol groups in cysteine residues, forming a covalent bond.
Common Reagents and Conditions:
Reagents: Iodoacetamide, rhodamine, sodium hydroxide.
Conditions: The reaction is typically carried out in an aqueous solution at room temperature.
Major Products: The major product of the reaction between this compound and cysteine-containing proteins is a covalently labeled protein, which can be visualized using fluorescence microscopy.
Scientific Research Applications
Rho-IA has a wide range of applications in scientific research:
Chemistry: Used as a fluorescent probe to study the structure and dynamics of proteins.
Biology: Facilitates the visualization of protein localization and interactions within cells.
Medicine: Aids in the development of diagnostic tools and therapeutic agents by enabling the study of protein functions and interactions.
Industry: Utilized in the development of biosensors and other analytical tools.
Comparison with Similar Compounds
Fluorescein-5-maleimide: Another fluorescent probe used for labeling cysteine residues.
Tetramethylrhodamine-5-maleimide: Similar to Rho-IA but with a different reactive group.
Uniqueness of this compound: this compound is unique due to its high specificity for cysteine residues and its strong fluorescence, which makes it an excellent tool for studying protein structures and functions in various biological contexts.
Properties
CAS No. |
114458-99-0 |
---|---|
Molecular Formula |
C26H24IN3O4 |
Molecular Weight |
569.39 |
IUPAC Name |
9-[2-Carboxy-4-[(2-iodoacetyl)amino]phenyl]-3,6-bis(dimethylamino)-xanthylium inner salt |
InChI |
InChI=1S/C26H24IN3O4/c1-29(2)16-6-9-19-22(12-16)34-23-13-17(30(3)4)7-10-20(23)25(19)18-8-5-15(28-24(31)14-27)11-21(18)26(32)33/h5-13H,14H2,1-4H3,(H-,28,31,32,33) |
InChI Key |
BCQMPZPXKLZJFQ-UHFFFAOYSA-N |
SMILES |
CN(C1=CC2=[O+]C3=C(C=CC(N(C)C)=C3)C(C4=CC=C(NC(CI)=O)C=C4C([O-])=O)=C2C=C1)C |
Appearance |
Solid powder |
Purity |
>98% (or refer to the Certificate of Analysis) |
shelf_life |
>2 years if stored properly |
solubility |
Soluble in DMSO |
storage |
Dry, dark and at 0 - 4 C for short term (days to weeks) or -20 C for long term (months to years). |
Synonyms |
Rho-IA; Rho IA; RhoIA; 5-TMRIA; Tetramethylrhodamine-4-iodoacetamide; 5TMRIA; 5 TMRIA; |
Origin of Product |
United States |
Retrosynthesis Analysis
AI-Powered Synthesis Planning: Our tool employs the Template_relevance Pistachio, Template_relevance Bkms_metabolic, Template_relevance Pistachio_ringbreaker, Template_relevance Reaxys, Template_relevance Reaxys_biocatalysis model, leveraging a vast database of chemical reactions to predict feasible synthetic routes.
One-Step Synthesis Focus: Specifically designed for one-step synthesis, it provides concise and direct routes for your target compounds, streamlining the synthesis process.
Accurate Predictions: Utilizing the extensive PISTACHIO, BKMS_METABOLIC, PISTACHIO_RINGBREAKER, REAXYS, REAXYS_BIOCATALYSIS database, our tool offers high-accuracy predictions, reflecting the latest in chemical research and data.
Strategy Settings
Precursor scoring | Relevance Heuristic |
---|---|
Min. plausibility | 0.01 |
Model | Template_relevance |
Template Set | Pistachio/Bkms_metabolic/Pistachio_ringbreaker/Reaxys/Reaxys_biocatalysis |
Top-N result to add to graph | 6 |
Feasible Synthetic Routes
Disclaimer and Information on In-Vitro Research Products
Please be aware that all articles and product information presented on BenchChem are intended solely for informational purposes. The products available for purchase on BenchChem are specifically designed for in-vitro studies, which are conducted outside of living organisms. In-vitro studies, derived from the Latin term "in glass," involve experiments performed in controlled laboratory settings using cells or tissues. It is important to note that these products are not categorized as medicines or drugs, and they have not received approval from the FDA for the prevention, treatment, or cure of any medical condition, ailment, or disease. We must emphasize that any form of bodily introduction of these products into humans or animals is strictly prohibited by law. It is essential to adhere to these guidelines to ensure compliance with legal and ethical standards in research and experimentation.