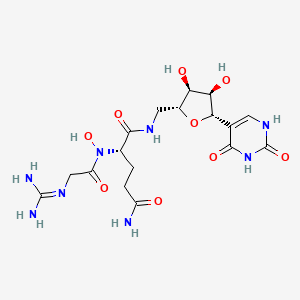
Pseudouridimycin
Overview
Description
Pseudouridimycin is a natural product that was discovered by screening microbial fermentation extracts for inhibitors of ribonucleic acid polymerase. It is known for its antibacterial properties and its ability to inhibit ribonucleic acid polymerase, making it a promising compound for scientific research and potential therapeutic applications .
Mechanism of Action
Target of Action
Pseudouridimycin primarily targets bacterial RNA polymerase (RNAP), an enzyme responsible for bacterial RNA synthesis . This enzyme plays a crucial role in the transcription process, where it catalyzes the synthesis of RNA from DNA.
Mode of Action
This compound inhibits bacterial RNA polymerase through a binding site and mechanism that are different from those of clinically approved RNA polymerase inhibitors of the rifamycin and lipiarmycin classes . This compound functions as a nucleoside-analog inhibitor of bacterial RNA polymerase, meaning that it mimics a nucleoside-triphosphate (NTP), the chemical “building block” that bacterial RNA polymerase uses to synthesize RNA . By occupying the NTP binding site, this compound prevents NTPs from binding, thereby inhibiting the function of the bacterial RNA polymerase .
Biochemical Pathways
The biosynthesis of this compound proceeds through the formation of pseudouridine by the pseudouridine synthase PumJ, with specialized oxidoreductase, aminotransferase, and amide ligases leading to the final compound
Result of Action
The result of this compound’s action is the inhibition of bacterial RNA synthesis, which leads to the death of the bacteria . It has been shown to be effective against a broad spectrum of drug-sensitive and drug-resistant bacteria in a test tube and can cure bacterial infections in mice .
Action Environment
The action of this compound is influenced by the environment within the bacterial cell where the RNA polymerase is located. It is worth noting that this compound was discovered by screening microbial fermentation extracts for RNA polymerase inhibitors , suggesting that it is produced in response to certain environmental conditions within the microbial community.
Biochemical Analysis
Biochemical Properties
Pseudouridimycin functions as a nucleoside-analog inhibitor that competes with uridine triphosphate for occupancy of the RNA polymerase nucleotide addition site . It interacts with bacterial RNA polymerase through a binding site and mechanism distinct from those of clinically approved RNA polymerase inhibitors such as rifamycin and lipiarmycin . The interaction involves the inhibition of nucleotide addition, with high concentrations of uridine triphosphate overcoming the transcription inhibition by this compound . This selective inhibition is crucial for its antibacterial properties, as it targets bacterial RNA polymerase without affecting human RNA polymerase .
Cellular Effects
This compound exerts its effects on bacterial cells by inhibiting RNA synthesis, which is essential for bacterial growth and replication . This inhibition leads to a cessation of bacterial cell division and ultimately cell death. The compound has shown activity against both Gram-positive and Gram-negative bacteria, making it a versatile antibacterial agent . Additionally, this compound’s selective inhibition of bacterial RNA polymerase ensures minimal impact on human cells, reducing potential side effects .
Molecular Mechanism
At the molecular level, this compound binds to the RNA polymerase nucleotide addition site, competing with uridine triphosphate . This binding prevents the incorporation of uridine into the growing RNA chain, effectively halting transcription . The compound’s unique binding interactions and selective inhibition of bacterial RNA polymerase are attributed to differences in the active site residues between bacterial and human RNA polymerases . These differences result in favorable binding interactions with bacterial RNA polymerase and unfavorable interactions with human RNA polymerase, ensuring selective inhibition .
Temporal Effects in Laboratory Settings
In laboratory settings, this compound has demonstrated stability and efficacy over time. Studies have shown that the compound maintains its antibacterial activity with a low rate of resistance emergence . Chemical stability studies revealed that this compound undergoes intramolecular hydroxamate bond scission as a major decomposition pathway in aqueous buffer . Replacement of the hydroxamate bond with a tertiary amide has been explored to improve stability and biological activity .
Dosage Effects in Animal Models
In animal models, this compound has shown efficacy in clearing bacterial infections. For instance, in a mouse Streptococcus pyogenes peritonitis model, this compound demonstrated effective infection clearance with median effective doses of approximately 9 mg/kg upon intravenous administration and 30 mg/kg upon subcutaneous administration . These studies highlight the compound’s potential for in vivo efficacy and tolerability in mammals .
Metabolic Pathways
This compound is involved in metabolic pathways that include the production of free pseudouridine by the dedicated pseudouridine synthase, PumJ . The biosynthesis pathway involves specialized oxidoreductases, aminotransferases, and amide ligases that contribute to the formation of the final compound . These enzymes and cofactors play a crucial role in the metabolic flux and levels of metabolites associated with this compound biosynthesis .
Transport and Distribution
Within cells and tissues, this compound is transported and distributed through interactions with specific transporters and binding proteins . These interactions influence the compound’s localization and accumulation, ensuring its effective delivery to target sites . The selective transport and distribution mechanisms contribute to the compound’s antibacterial efficacy and reduced toxicity .
Subcellular Localization
This compound’s subcellular localization is directed by targeting signals and post-translational modifications that guide it to specific compartments or organelles . These localization mechanisms are essential for the compound’s activity and function, ensuring that it reaches the bacterial RNA polymerase to exert its inhibitory effects . The precise subcellular targeting enhances the compound’s selectivity and minimizes off-target effects .
Preparation Methods
The preparation of pseudouridimycin involves microbial fermentation. The specific strain used for fermentation is typically a type of bacteria that produces the compound naturally. The fermentation process involves growing the bacteria in a nutrient-rich medium, followed by extraction and purification of the compound from the fermentation broth .
Chemical Reactions Analysis
Pseudouridimycin undergoes various chemical reactions, including:
Oxidation: This reaction involves the loss of electrons from this compound, often facilitated by oxidizing agents.
Reduction: This reaction involves the gain of electrons, typically using reducing agents.
Substitution: This reaction involves the replacement of one functional group in this compound with another. Common reagents for these reactions include halogens and nucleophiles.
Hydrolysis: This reaction involves the breaking of chemical bonds in this compound by the addition of water.
The major products formed from these reactions depend on the specific conditions and reagents used .
Scientific Research Applications
Scientific Research Applications
Pseudouridimycin has several key applications across various fields:
Antibiotic Development
- Potential Therapeutic Agent : PUM has demonstrated efficacy against multidrug-resistant bacteria, making it a promising candidate for new antibiotic therapies. Studies have shown that it effectively inhibits macrolide-resistant Streptococcus pyogenes at low concentrations (0.1–1 μg/mL) .
- In Vivo Efficacy : In animal models, PUM has been shown to clear infections caused by S. pyogenes, with effective doses ranging from 9 mg/kg to 30 mg/kg depending on the administration route .
Mechanistic Studies
- Understanding RNAP Inhibition : PUM serves as a model compound for studying the mechanisms underlying RNA polymerase inhibition. Its unique binding interactions provide insights into how nucleoside analogs can be designed to target bacterial transcription processes effectively .
- Resistance Mechanisms : Research into PUM's low resistance rates compared to other antibiotics offers valuable information for developing strategies to combat antibiotic resistance in clinical settings .
Biosynthetic Pathway Exploration
- Biosynthesis Insights : The study of PUM's biosynthetic pathway reveals novel features of C-nucleoside antibiotics. Researchers have identified key enzymes involved in its synthesis, such as pseudouridine synthase PumJ, which may lead to the discovery of additional antibiotics with similar structures and functions .
- Metabolomic Studies : Analyses of Streptomyces sp. ID38640, the producer of PUM, have uncovered other metabolites and potential antibiotic compounds, expanding the scope of natural product research .
Antibacterial Activity of this compound
Bacterial Strain | Minimum Inhibitory Concentration (MIC) | Resistance Status |
---|---|---|
Streptococcus pyogenes | 0.1–1 μg/mL | Macrolide-resistant |
Staphylococcus aureus | 4–6 μg/mL | Multidrug-resistant |
Escherichia coli | 2–12 μg/mL | Drug-sensitive |
Summary of Key Research Findings
Case Study 1: Efficacy Against Multidrug-Resistant Strains
A study evaluated the effectiveness of PUM against various strains of S. pyogenes, including those resistant to erythromycin. Results indicated that PUM maintained its antibacterial activity at significantly lower concentrations than previously reported, suggesting potential for clinical application in treating resistant infections .
Case Study 2: Biosynthetic Pathway Analysis
Research on the biosynthesis of PUM revealed unexpected features and led to the identification of additional metabolites produced by Streptomyces sp. ID38640. This work not only elucidated the biosynthetic route for PUM but also opened avenues for discovering new antibiotics from related pathways .
Comparison with Similar Compounds
Pseudouridimycin is unique in its ability to inhibit ribonucleic acid polymerase. Similar compounds include:
Rifampicin: Another ribonucleic acid polymerase inhibitor, but with a different binding site and mechanism of action.
Streptolydigin: Inhibits ribonucleic acid polymerase by binding to a different site on the enzyme.
Myxopyronin: Inhibits ribonucleic acid polymerase by preventing the formation of the transcription initiation complex.
This compound is unique in its structure and binding mechanism, making it a valuable tool for studying ribonucleic acid polymerase and developing new antibacterial agents .
Biological Activity
Pseudouridimycin (PUM) is a novel antibiotic with significant biological activity against a range of bacterial pathogens, particularly those resistant to conventional antibiotics. This article delves into its mechanisms of action, efficacy, and potential applications, supported by relevant data tables and case studies.
Overview of this compound
PUM is a C-nucleoside analogue produced by certain Streptomyces species. It functions primarily as an inhibitor of bacterial RNA polymerase (RNAP), specifically targeting the nucleotide addition site. This unique mechanism differentiates it from other RNAP inhibitors such as rifamycins and lipiarmycins, which bind to different sites on the enzyme .
PUM mimics uridine triphosphate (UTP) and competes for binding at the RNAP active center. Its structure allows it to form hydrogen bonds with both the DNA template and the nascent RNA product, effectively blocking transcription. The binding affinity of PUM has been quantified, showing an IC50 of approximately 0.1 μM and a minimum inhibitory concentration (MIC) ranging from 4 to 6 μg/mL against various bacterial strains .
Efficacy Against Bacterial Strains
PUM exhibits broad-spectrum antibacterial activity against both Gram-positive and Gram-negative bacteria. Notably, it has shown effectiveness against drug-resistant strains, including Streptococcus pyogenes. The following table summarizes its activity against various bacterial strains:
Bacterial Strain | MIC (μg/mL) | Resistance Type |
---|---|---|
Streptococcus pyogenes | 0.1 - 1 | Erythromycin-resistant |
Escherichia coli | 4 - 6 | Multidrug-resistant |
Staphylococcus aureus | 2 - 8 | Methicillin-resistant |
Klebsiella pneumoniae | 6 - 12 | Extended-spectrum beta-lactamase (ESBL) producing |
Table 1: Antibacterial activity of this compound against various bacterial strains.
Study on Drug-Resistant S. pyogenes
In a recent study, PUM was tested against clinical isolates of erythromycin-resistant S. pyogenes. The results indicated that PUM not only inhibited these resistant strains but also demonstrated bactericidal activity in vivo, effectively clearing infections in mouse models .
Mechanistic Insights from Structural Studies
Structural studies have identified specific binding interactions between PUM and the RNAP subunits in S. pyogenes. The N-terminal domain of the β′ subunit was pinpointed as a critical target for PUM binding, providing insights into its selective inhibition mechanism .
Biosynthesis Pathway
The biosynthetic pathway for PUM involves several key enzymes, including the pseudouridine synthase PumJ, which plays a central role in its synthesis. Recent genomic analyses have revealed that this pathway is conserved across various Streptomyces species, indicating a potential for engineered production of PUM analogs .
Resistance Profile
One of the notable features of PUM is its low rate of resistance acquisition compared to traditional antibiotics. Studies indicate that spontaneous resistance rates are less than one-tenth that observed with rifamycins and lipiarmycins . This characteristic makes PUM a promising candidate for further development as an antibiotic.
Properties
IUPAC Name |
(2S)-2-[[2-(diaminomethylideneamino)acetyl]-hydroxyamino]-N-[[(2R,3S,4R,5S)-5-(2,4-dioxo-1H-pyrimidin-5-yl)-3,4-dihydroxyoxolan-2-yl]methyl]pentanediamide | |
---|---|---|
Source | PubChem | |
URL | https://pubchem.ncbi.nlm.nih.gov | |
Description | Data deposited in or computed by PubChem | |
InChI |
InChI=1S/C17H26N8O9/c18-9(26)2-1-7(25(33)10(27)5-22-16(19)20)15(31)21-4-8-11(28)12(29)13(34-8)6-3-23-17(32)24-14(6)30/h3,7-8,11-13,28-29,33H,1-2,4-5H2,(H2,18,26)(H,21,31)(H4,19,20,22)(H2,23,24,30,32)/t7-,8+,11+,12+,13-/m0/s1 | |
Source | PubChem | |
URL | https://pubchem.ncbi.nlm.nih.gov | |
Description | Data deposited in or computed by PubChem | |
InChI Key |
XDEYHXABZOKKDZ-YFKLLHAASA-N | |
Source | PubChem | |
URL | https://pubchem.ncbi.nlm.nih.gov | |
Description | Data deposited in or computed by PubChem | |
Canonical SMILES |
C1=C(C(=O)NC(=O)N1)C2C(C(C(O2)CNC(=O)C(CCC(=O)N)N(C(=O)CN=C(N)N)O)O)O | |
Source | PubChem | |
URL | https://pubchem.ncbi.nlm.nih.gov | |
Description | Data deposited in or computed by PubChem | |
Isomeric SMILES |
C1=C(C(=O)NC(=O)N1)[C@H]2[C@@H]([C@@H]([C@H](O2)CNC(=O)[C@H](CCC(=O)N)N(C(=O)CN=C(N)N)O)O)O | |
Source | PubChem | |
URL | https://pubchem.ncbi.nlm.nih.gov | |
Description | Data deposited in or computed by PubChem | |
Molecular Formula |
C17H26N8O9 | |
Source | PubChem | |
URL | https://pubchem.ncbi.nlm.nih.gov | |
Description | Data deposited in or computed by PubChem | |
Molecular Weight |
486.4 g/mol | |
Source | PubChem | |
URL | https://pubchem.ncbi.nlm.nih.gov | |
Description | Data deposited in or computed by PubChem | |
CAS No. |
1566586-52-4 | |
Record name | Pseudouridimycin | |
Source | ChemIDplus | |
URL | https://pubchem.ncbi.nlm.nih.gov/substance/?source=chemidplus&sourceid=1566586524 | |
Description | ChemIDplus is a free, web search system that provides access to the structure and nomenclature authority files used for the identification of chemical substances cited in National Library of Medicine (NLM) databases, including the TOXNET system. | |
Disclaimer and Information on In-Vitro Research Products
Please be aware that all articles and product information presented on BenchChem are intended solely for informational purposes. The products available for purchase on BenchChem are specifically designed for in-vitro studies, which are conducted outside of living organisms. In-vitro studies, derived from the Latin term "in glass," involve experiments performed in controlled laboratory settings using cells or tissues. It is important to note that these products are not categorized as medicines or drugs, and they have not received approval from the FDA for the prevention, treatment, or cure of any medical condition, ailment, or disease. We must emphasize that any form of bodily introduction of these products into humans or animals is strictly prohibited by law. It is essential to adhere to these guidelines to ensure compliance with legal and ethical standards in research and experimentation.