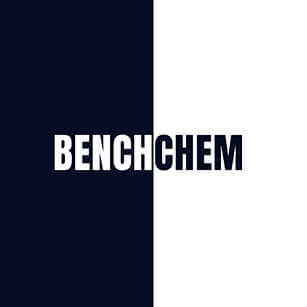
Proxyfan Oxalate
- Click on QUICK INQUIRY to receive a quote from our team of experts.
- With the quality product at a COMPETITIVE price, you can focus more on your research.
Overview
Description
Proxyfan is a histamine H3 receptor ligand known for its unique ability to act as a protean agonist. This means it can produce a range of effects, from full agonist to antagonist to inverse agonist, depending on the tissue and the level of constitutive activity of the histamine H3 receptor . This complex activity profile makes Proxyfan a valuable tool in scientific research.
Preparation Methods
The synthesis of Proxyfan involves several steps. The preferred IUPAC name for Proxyfan is 4-[3-(Benzyloxy)propyl]-1H-imidazole. The synthetic route typically involves the reaction of 4-(3-bromopropyl)-1H-imidazole with benzyl alcohol under basic conditions to form the desired product
Chemical Reactions Analysis
Proxyfan undergoes various chemical reactions, including:
Oxidation: Proxyfan can be oxidized under specific conditions, although detailed studies on this are limited.
Substitution: Proxyfan can undergo substitution reactions, particularly at the imidazole ring and the benzyloxy group.
Common reagents and conditions used in these reactions include strong bases for substitution reactions and oxidizing agents for oxidation reactions. The major products formed from these reactions depend on the specific conditions and reagents used.
Scientific Research Applications
Proxyfan has a wide range of scientific research applications:
Chemistry: Proxyfan is used as a tool to study the pharmacology of histamine H3 receptors.
Medicine: Proxyfan has been studied for its potential therapeutic effects in conditions such as obesity and diabetes.
Industry: While its industrial applications are limited, Proxyfan’s unique pharmacological profile makes it a valuable compound for drug development and research.
Mechanism of Action
Proxyfan exerts its effects through its interaction with histamine H3 receptors. As a protean agonist, it can act as a full agonist, antagonist, or inverse agonist depending on the tissue and the level of constitutive activity of the receptor . This allows Proxyfan to modulate the release of histamine and other neurotransmitters, influencing various physiological processes such as appetite, cognition, and arousal .
Comparison with Similar Compounds
Proxyfan is unique among histamine H3 receptor ligands due to its protean agonist activity. Similar compounds include:
Thioperamide: An inverse agonist at histamine H3 receptors, used to study the role of these receptors in various physiological processes.
Imetit: A full agonist at histamine H3 receptors, used to investigate the effects of receptor activation.
Proxyfan’s ability to produce a range of effects depending on the tissue and receptor activity level sets it apart from these other compounds, making it a versatile tool in scientific research.
Properties
CAS No. |
177708-09-7 |
---|---|
Molecular Formula |
C13H16N2O |
Molecular Weight |
216.28 g/mol |
IUPAC Name |
5-(3-phenylmethoxypropyl)-1H-imidazole |
InChI |
InChI=1S/C13H16N2O/c1-2-5-12(6-3-1)10-16-8-4-7-13-9-14-11-15-13/h1-3,5-6,9,11H,4,7-8,10H2,(H,14,15) |
InChI Key |
WNWALBVQAAIULR-UHFFFAOYSA-N |
SMILES |
O=C(O)C(O)=O.C1(CCCOCC2=CC=CC=C2)=CNC=N1 |
Canonical SMILES |
C1=CC=C(C=C1)COCCCC2=CN=CN2 |
Appearance |
Solid powder |
Purity |
>98% (or refer to the Certificate of Analysis) |
shelf_life |
>2 years if stored properly |
solubility |
Soluble in DMSO |
storage |
Dry, dark and at 0 - 4 C for short term (days to weeks) or -20 C for long term (months to years). |
Synonyms |
Proxyfan Oxalate |
Origin of Product |
United States |
Retrosynthesis Analysis
AI-Powered Synthesis Planning: Our tool employs the Template_relevance Pistachio, Template_relevance Bkms_metabolic, Template_relevance Pistachio_ringbreaker, Template_relevance Reaxys, Template_relevance Reaxys_biocatalysis model, leveraging a vast database of chemical reactions to predict feasible synthetic routes.
One-Step Synthesis Focus: Specifically designed for one-step synthesis, it provides concise and direct routes for your target compounds, streamlining the synthesis process.
Accurate Predictions: Utilizing the extensive PISTACHIO, BKMS_METABOLIC, PISTACHIO_RINGBREAKER, REAXYS, REAXYS_BIOCATALYSIS database, our tool offers high-accuracy predictions, reflecting the latest in chemical research and data.
Strategy Settings
Precursor scoring | Relevance Heuristic |
---|---|
Min. plausibility | 0.01 |
Model | Template_relevance |
Template Set | Pistachio/Bkms_metabolic/Pistachio_ringbreaker/Reaxys/Reaxys_biocatalysis |
Top-N result to add to graph | 6 |
Feasible Synthetic Routes
Disclaimer and Information on In-Vitro Research Products
Please be aware that all articles and product information presented on BenchChem are intended solely for informational purposes. The products available for purchase on BenchChem are specifically designed for in-vitro studies, which are conducted outside of living organisms. In-vitro studies, derived from the Latin term "in glass," involve experiments performed in controlled laboratory settings using cells or tissues. It is important to note that these products are not categorized as medicines or drugs, and they have not received approval from the FDA for the prevention, treatment, or cure of any medical condition, ailment, or disease. We must emphasize that any form of bodily introduction of these products into humans or animals is strictly prohibited by law. It is essential to adhere to these guidelines to ensure compliance with legal and ethical standards in research and experimentation.