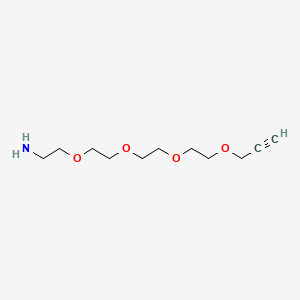
Propargyl-PEG4-amine
Overview
Description
Propargyl-PEG4-amine is a polyethylene glycol derivative that contains both a propargyl group and an amine group. The amine group is reactive with carboxylic acids, activated N-hydroxysuccinimide esters, and carbonyl groups such as ketones and aldehydes. . This compound is widely used in bioconjugation, drug delivery, and other biomedical applications due to its versatility and reactivity.
Mechanism of Action
Target of Action
Propargyl-PEG4-amine is a PEG-based PROTAC linker . It is used in the synthesis of PROTACs (Proteolysis-Targeting Chimeras), which are molecules designed to degrade specific proteins within cells . The primary targets of this compound are proteins that are marked for degradation by the PROTACs it helps form .
Mode of Action
This compound contains an Alkyne group, which can undergo copper-catalyzed azide-alkyne cycloaddition (CuAAC) with molecules containing Azide groups . This reaction is a key step in the formation of PROTACs . The resulting PROTACs contain two different ligands connected by a linker; one is a ligand for an E3 ubiquitin ligase and the other is for the target protein . The PROTACs exploit the intracellular ubiquitin-proteasome system to selectively degrade target proteins .
Biochemical Pathways
The biochemical pathways affected by this compound are those involved in protein degradation . By facilitating the formation of PROTACs, this compound indirectly influences the ubiquitin-proteasome system, a major pathway for protein degradation in cells . The downstream effects include the degradation of specific target proteins, which can have various effects depending on the functions of these proteins .
Pharmacokinetics
As a peg-based compound, it is expected to have good solubility and stability, which can enhance its bioavailability .
Result of Action
The primary result of the action of this compound is the formation of PROTACs that can selectively degrade specific proteins within cells . The degradation of these proteins can influence various cellular processes, depending on the roles of the target proteins .
Action Environment
The action of this compound, like many other chemical reactions, can be influenced by various environmental factors. As a PEG-based compound, it is expected to have good stability .
Biochemical Analysis
Biochemical Properties
Propargyl-PEG4-amine plays a significant role in biochemical reactions, primarily through its involvement in click chemistry. The amine group of this compound is reactive with carboxylic acids, activated NHS esters, and carbonyls (ketones and aldehydes). The propargyl group can react with azide-bearing compounds or biomolecules via CuAAC to yield a stable triazole linkage . This reaction is highly specific and efficient, allowing for the precise modification of proteins, enzymes, and other biomolecules. This compound is often used to label proteins and peptides, facilitating their detection and analysis in various biochemical assays.
Cellular Effects
This compound influences various cellular processes by modifying biomolecules within the cell. It can affect cell signaling pathways, gene expression, and cellular metabolism by covalently attaching to target proteins and altering their function. For example, this compound can be used to label and track specific proteins within cells, providing insights into their localization and interactions. Additionally, the modification of enzymes with this compound can impact their catalytic activity, leading to changes in metabolic pathways and cellular functions .
Molecular Mechanism
The molecular mechanism of this compound involves its ability to form covalent bonds with target biomolecules through click chemistry. The propargyl group of this compound reacts with azide groups on biomolecules in the presence of a copper catalyst, forming a stable triazole linkage. This covalent modification can alter the structure and function of the target biomolecule, leading to changes in its activity and interactions. For example, the modification of enzymes with this compound can result in enzyme inhibition or activation, depending on the site of modification and the nature of the enzyme .
Temporal Effects in Laboratory Settings
In laboratory settings, the effects of this compound can change over time due to its stability and degradation. This compound is generally stable under standard laboratory conditions, but its reactivity can decrease over time if not stored properly. Long-term studies have shown that this compound can have lasting effects on cellular function, particularly when used to modify key regulatory proteins and enzymes. These modifications can lead to sustained changes in cell signaling pathways and metabolic processes .
Dosage Effects in Animal Models
The effects of this compound in animal models vary with different dosages. At low doses, this compound can effectively label and modify target biomolecules without causing significant toxicity. At higher doses, this compound may exhibit toxic effects, including cellular stress and apoptosis. Studies have shown that there is a threshold dose above which the adverse effects of this compound become more pronounced, highlighting the importance of optimizing dosage for specific applications .
Metabolic Pathways
This compound is involved in various metabolic pathways through its interactions with enzymes and other biomolecules. The modification of enzymes with this compound can alter their catalytic activity, leading to changes in metabolic flux and metabolite levels. For example, the inhibition of key metabolic enzymes by this compound can result in the accumulation or depletion of specific metabolites, affecting overall cellular metabolism .
Transport and Distribution
Within cells and tissues, this compound is transported and distributed through interactions with transporters and binding proteins. These interactions can influence the localization and accumulation of this compound, affecting its activity and function. For example, this compound may be transported into specific cellular compartments or organelles, where it can modify target biomolecules and exert its effects .
Subcellular Localization
The subcellular localization of this compound is determined by its interactions with targeting signals and post-translational modifications. This compound can be directed to specific compartments or organelles within the cell, where it can modify target proteins and enzymes. This localization is crucial for its activity, as it allows this compound to exert its effects in specific cellular contexts. For example, the modification of mitochondrial proteins by this compound can impact mitochondrial function and energy metabolism .
Preparation Methods
Synthetic Routes and Reaction Conditions: Propargyl-PEG4-amine can be synthesized through various methods. One common approach involves the modification of commercially available polyethylene glycol derivatives. For instance, a bifunctional polyethylene glycol with an α-hydroxyl group and an ω-carboxyl group can be used as the starting material. The carboxyl group is modified into a propargyl group, while the hydroxyl group can be further modified into other functional groups such as carboxyl, mercapto, or hydrazide .
Industrial Production Methods: Industrial production of this compound typically involves large-scale synthesis using optimized reaction conditions to ensure high yield and purity. The process may include the use of catalysts and specific reaction conditions to facilitate the formation of the desired product. For example, the use of a novel magnetically reusable manganese nanocatalyst has been reported to efficiently catalyze the synthesis of propargyl amines through three-component coupling reactions .
Chemical Reactions Analysis
Types of Reactions: Propargyl-PEG4-amine undergoes various chemical reactions, including:
Substitution Reactions: The amine group can react with activated N-hydroxysuccinimide esters and carboxylic acids to form stable amide bonds.
Click Chemistry: The propargyl group can react with azide-bearing compounds via copper-catalyzed azide-alkyne cycloaddition to form stable triazole linkages.
Reductive Amination: The amine group can react with carbonyl groups such as ketones and aldehydes under reductive amination conditions.
Common Reagents and Conditions:
Copper Catalysts: Used in click chemistry reactions to facilitate the formation of triazole linkages.
Activated N-hydroxysuccinimide Esters: React with the amine group to form amide bonds.
Reducing Agents: Used in reductive amination reactions to convert carbonyl groups into amines.
Major Products:
Triazole Linkages: Formed through click chemistry reactions.
Amide Bonds: Formed through reactions with activated N-hydroxysuccinimide esters and carboxylic acids.
Scientific Research Applications
Propargyl-PEG4-amine has a wide range of applications in scientific research, including:
Chemistry: Used as a building block for the synthesis of more complex molecules and intermediates.
Biology: Employed in bioconjugation techniques to attach biomolecules to surfaces or other molecules.
Medicine: Utilized in drug delivery systems to improve the solubility and stability of therapeutic agents.
Industry: Applied in the development of advanced materials and surface modifications.
Comparison with Similar Compounds
Propargyl-PEG4-acid: Contains a carboxylic acid group instead of an amine group.
Propargylamine: A simpler compound with only a propargyl group and an amine group.
Uniqueness: Propargyl-PEG4-amine is unique due to its bifunctional nature, allowing it to participate in a wide range of chemical reactions. The presence of both a propargyl group and an amine group makes it highly versatile for use in bioconjugation, drug delivery, and other biomedical applications .
Biological Activity
Propargyl-PEG4-amine is a bifunctional polyethylene glycol (PEG) derivative that contains a propargyl group and an amine group. This compound has garnered attention in the fields of bioconjugation, drug delivery, and biomedical applications due to its unique chemical properties and biological activities. This article provides a comprehensive overview of the biological activity of this compound, including its mechanisms of action, biochemical pathways, and relevant case studies.
Overview of this compound
This compound is characterized by its ability to participate in click chemistry reactions, particularly the copper-catalyzed azide-alkyne cycloaddition (CuAAC). This reaction allows for the formation of stable triazole linkages, which are crucial for bioconjugation applications. The amine group in this compound can react with various electrophiles, such as carboxylic acids and activated N-hydroxysuccinimide (NHS) esters, facilitating the attachment of biomolecules .
Target of Action:
this compound primarily acts as a linker in proteolysis-targeting chimeras (PROTACs), which are designed to selectively degrade specific proteins within cells. The propargyl group allows for covalent modification of target proteins through click chemistry, altering their function and interactions.
Biochemical Pathways:
The compound influences several cellular processes by modifying biomolecules. Its ability to form covalent bonds can impact cell signaling pathways, gene expression, and metabolic processes. For example, it can be used to label proteins for tracking their localization and interactions within cells.
Pharmacokinetics:
As a PEG-based compound, this compound exhibits good solubility and stability, enhancing its bioavailability. This characteristic is essential for effective drug delivery systems where prolonged circulation time in biological systems is desired.
Cellular Effects
This compound modifies cellular functions by covalently attaching to target proteins. This modification can lead to:
- Altered Enzyme Activity: Depending on the site of modification, it can inhibit or activate enzymes, thereby influencing metabolic pathways.
- Changes in Protein Interactions: By attaching to specific proteins, it can alter their interactions with other biomolecules, impacting signaling pathways.
In laboratory settings, the temporal effects of this compound have shown that modifications may lead to sustained changes in cellular functions over time.
Case Studies and Research Findings
- Bioconjugation Applications:
-
Neuroprotective Studies:
- While not directly linked to this compound itself, related propargylamines have shown neuroprotective properties in various models. For instance, derivatives have been studied for their ability to reduce neuroinflammation and protect neuronal cells from oxidative stress . These findings suggest potential therapeutic applications for this compound in neurodegenerative diseases.
-
Protein Degradation via PROTACs:
- Research has illustrated that compounds like this compound serve as effective linkers in PROTACs designed for targeted protein degradation. These PROTACs utilize the unique reactivity of the propargyl group to engage with E3 ligases, leading to the ubiquitination and subsequent degradation of target proteins .
Comparison with Similar Compounds
Compound Name | Functional Groups | Unique Features |
---|---|---|
Propargyl-PEG4-acid | Carboxylic acid | Lacks amine functionality |
Propargylamine | Amine and propargyl | Simpler structure with limited versatility |
Propargyl-PEG6-amine | Longer PEG chain | Improved solubility but similar reactivity |
This compound stands out due to its bifunctional nature, allowing diverse chemical reactions essential for bioconjugation and drug delivery applications.
Properties
IUPAC Name |
2-[2-[2-(2-prop-2-ynoxyethoxy)ethoxy]ethoxy]ethanamine | |
---|---|---|
Source | PubChem | |
URL | https://pubchem.ncbi.nlm.nih.gov | |
Description | Data deposited in or computed by PubChem | |
InChI |
InChI=1S/C11H21NO4/c1-2-4-13-6-8-15-10-11-16-9-7-14-5-3-12/h1H,3-12H2 | |
Source | PubChem | |
URL | https://pubchem.ncbi.nlm.nih.gov | |
Description | Data deposited in or computed by PubChem | |
InChI Key |
QDLPAHLHHBCWOW-UHFFFAOYSA-N | |
Source | PubChem | |
URL | https://pubchem.ncbi.nlm.nih.gov | |
Description | Data deposited in or computed by PubChem | |
Canonical SMILES |
C#CCOCCOCCOCCOCCN | |
Source | PubChem | |
URL | https://pubchem.ncbi.nlm.nih.gov | |
Description | Data deposited in or computed by PubChem | |
Molecular Formula |
C11H21NO4 | |
Source | PubChem | |
URL | https://pubchem.ncbi.nlm.nih.gov | |
Description | Data deposited in or computed by PubChem | |
Molecular Weight |
231.29 g/mol | |
Source | PubChem | |
URL | https://pubchem.ncbi.nlm.nih.gov | |
Description | Data deposited in or computed by PubChem | |
CAS No. |
1013921-36-2 | |
Record name | 3,6,9,12-tetraoxapentadec-14-yn-1-amine | |
Source | European Chemicals Agency (ECHA) | |
URL | https://echa.europa.eu/information-on-chemicals | |
Description | The European Chemicals Agency (ECHA) is an agency of the European Union which is the driving force among regulatory authorities in implementing the EU's groundbreaking chemicals legislation for the benefit of human health and the environment as well as for innovation and competitiveness. | |
Explanation | Use of the information, documents and data from the ECHA website is subject to the terms and conditions of this Legal Notice, and subject to other binding limitations provided for under applicable law, the information, documents and data made available on the ECHA website may be reproduced, distributed and/or used, totally or in part, for non-commercial purposes provided that ECHA is acknowledged as the source: "Source: European Chemicals Agency, http://echa.europa.eu/". Such acknowledgement must be included in each copy of the material. ECHA permits and encourages organisations and individuals to create links to the ECHA website under the following cumulative conditions: Links can only be made to webpages that provide a link to the Legal Notice page. | |
Synthesis routes and methods
Procedure details
Disclaimer and Information on In-Vitro Research Products
Please be aware that all articles and product information presented on BenchChem are intended solely for informational purposes. The products available for purchase on BenchChem are specifically designed for in-vitro studies, which are conducted outside of living organisms. In-vitro studies, derived from the Latin term "in glass," involve experiments performed in controlled laboratory settings using cells or tissues. It is important to note that these products are not categorized as medicines or drugs, and they have not received approval from the FDA for the prevention, treatment, or cure of any medical condition, ailment, or disease. We must emphasize that any form of bodily introduction of these products into humans or animals is strictly prohibited by law. It is essential to adhere to these guidelines to ensure compliance with legal and ethical standards in research and experimentation.