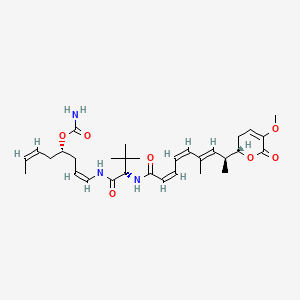
Plocabulin
Overview
Description
Plocabulin, also known as PM060184, is a novel marine-derived microtubule-binding agent. It was originally isolated from the marine sponge Lithoplocamia lithistoides. This compound has shown significant potential as an antitumor agent due to its ability to disrupt microtubule dynamics, which are essential for cell division and intracellular transport .
Scientific Research Applications
Plocabulin has a wide range of scientific research applications, including:
Chemistry: this compound serves as a valuable tool for studying microtubule dynamics and the effects of microtubule disruption on cellular processes.
Biology: In biological research, this compound is used to investigate the mechanisms of cell division and intracellular transport, as well as the role of microtubules in these processes.
Medicine: this compound is being explored as a potential therapeutic agent for the treatment of various cancers. .
Industry: In the pharmaceutical industry, this compound is being developed as a novel anticancer drug.
Mechanism of Action
Plocabulin inhibits angiogenesis by modulation of microtubule dynamics in endothelial cells . It inhibits microtubule dynamics in endothelial cells at picomolar concentrations . This rapid collapse of the endothelial tubular network in vitro occurs in a concentration-dependent manner and is observed at concentrations lower than that affecting cell survival .
Safety and Hazards
Plocabulin is toxic and contains a pharmaceutically active ingredient . Handling should only be performed by personnel trained and familiar with handling of potent active pharmaceutical ingredients . It is a moderate to severe irritant to the skin and eyes . It is very toxic if swallowed, irritating to skin, and has a risk of serious damages to eyes . It is toxic and has a danger of serious damage to health by prolonged exposure .
Future Directions
Plocabulin is currently undergoing phase II clinical trials . It has shown potent antitumor activity in patient-derived xenograft models of soft tissue sarcoma . The results demonstrate the in vivo efficacy of this compound in the preclinical models of soft tissue sarcoma and corroborate the findings of previous studies . These data provide a convincing rationale for further clinical exploration of this compound in soft tissue sarcomas .
Preparation Methods
Synthetic Routes and Reaction Conditions: Plocabulin is synthesized through a complex multi-step process that involves the total synthesis of its intricate molecular structureKey steps include cyclization, oxidation, and selective functional group modifications .
Industrial Production Methods: Industrial production of this compound involves optimizing the synthetic route to ensure high yield and purity. This often includes the use of advanced techniques such as high-performance liquid chromatography (HPLC) for purification and nuclear magnetic resonance (NMR) spectroscopy for structural confirmation. The process is designed to be scalable, allowing for the production of this compound in quantities sufficient for clinical and commercial use .
Chemical Reactions Analysis
Types of Reactions: Plocabulin undergoes various chemical reactions, including:
Oxidation: this compound can be oxidized to introduce or modify functional groups, enhancing its biological activity.
Reduction: Reduction reactions can be used to alter the oxidation state of specific atoms within the molecule, potentially modifying its pharmacokinetic properties.
Common Reagents and Conditions:
Oxidation: Common oxidizing agents include potassium permanganate and chromium trioxide.
Reduction: Reducing agents such as lithium aluminum hydride and sodium borohydride are frequently used.
Substitution: Reagents like alkyl halides and acyl chlorides are employed under controlled conditions to achieve the desired substitutions.
Major Products: The major products formed from these reactions include various derivatives of this compound with modified functional groups, which can exhibit different levels of biological activity and stability .
Comparison with Similar Compounds
Plocabulin is unique among microtubule-binding agents due to its marine origin and its specific binding site on tubulin. Similar compounds include:
Paclitaxel: A well-known microtubule-stabilizing agent used in cancer therapy. Unlike this compound, paclitaxel stabilizes microtubules rather than disrupting them.
Vinblastine: Another microtubule-disrupting agent that binds to a different site on tubulin compared to this compound.
Colchicine: A microtubule-disrupting agent that binds to tubulin but has a different mechanism of action and clinical application
This compound’s unique binding site and mechanism of action make it a valuable addition to the arsenal of microtubule-targeting agents, offering potential advantages in terms of efficacy and safety.
Properties
IUPAC Name |
[(1Z,4S,6Z)-1-[[(2S)-2-[[(2Z,4Z,6E,8S)-8-[(2S)-5-methoxy-6-oxo-2,3-dihydropyran-2-yl]-6-methylnona-2,4,6-trienoyl]amino]-3,3-dimethylbutanoyl]amino]octa-1,6-dien-4-yl] carbamate | |
---|---|---|
Source | PubChem | |
URL | https://pubchem.ncbi.nlm.nih.gov | |
Description | Data deposited in or computed by PubChem | |
InChI |
InChI=1S/C31H45N3O7/c1-8-9-14-23(40-30(32)38)15-12-19-33-28(36)27(31(4,5)6)34-26(35)16-11-10-13-21(2)20-22(3)24-17-18-25(39-7)29(37)41-24/h8-13,16,18-20,22-24,27H,14-15,17H2,1-7H3,(H2,32,38)(H,33,36)(H,34,35)/b9-8-,13-10-,16-11-,19-12-,21-20+/t22-,23-,24-,27+/m0/s1 | |
Source | PubChem | |
URL | https://pubchem.ncbi.nlm.nih.gov | |
Description | Data deposited in or computed by PubChem | |
InChI Key |
IEKGSKLKBICCHQ-BDOJOPHNSA-N | |
Source | PubChem | |
URL | https://pubchem.ncbi.nlm.nih.gov | |
Description | Data deposited in or computed by PubChem | |
Canonical SMILES |
CC=CCC(CC=CNC(=O)C(C(C)(C)C)NC(=O)C=CC=CC(=CC(C)C1CC=C(C(=O)O1)OC)C)OC(=O)N | |
Source | PubChem | |
URL | https://pubchem.ncbi.nlm.nih.gov | |
Description | Data deposited in or computed by PubChem | |
Isomeric SMILES |
C/C=C\C[C@@H](C/C=C\NC(=O)[C@H](C(C)(C)C)NC(=O)/C=C\C=C/C(=C/[C@H](C)[C@@H]1CC=C(C(=O)O1)OC)/C)OC(=O)N | |
Source | PubChem | |
URL | https://pubchem.ncbi.nlm.nih.gov | |
Description | Data deposited in or computed by PubChem | |
Molecular Formula |
C31H45N3O7 | |
Source | PubChem | |
URL | https://pubchem.ncbi.nlm.nih.gov | |
Description | Data deposited in or computed by PubChem | |
Molecular Weight |
571.7 g/mol | |
Source | PubChem | |
URL | https://pubchem.ncbi.nlm.nih.gov | |
Description | Data deposited in or computed by PubChem | |
CAS No. |
960210-99-5 | |
Record name | Plocabulin [INN] | |
Source | ChemIDplus | |
URL | https://pubchem.ncbi.nlm.nih.gov/substance/?source=chemidplus&sourceid=0960210995 | |
Description | ChemIDplus is a free, web search system that provides access to the structure and nomenclature authority files used for the identification of chemical substances cited in National Library of Medicine (NLM) databases, including the TOXNET system. | |
Record name | PM-060184 | |
Source | DrugBank | |
URL | https://www.drugbank.ca/drugs/DB13137 | |
Description | The DrugBank database is a unique bioinformatics and cheminformatics resource that combines detailed drug (i.e. chemical, pharmacological and pharmaceutical) data with comprehensive drug target (i.e. sequence, structure, and pathway) information. | |
Explanation | Creative Common's Attribution-NonCommercial 4.0 International License (http://creativecommons.org/licenses/by-nc/4.0/legalcode) | |
Record name | PLOCABULIN | |
Source | FDA Global Substance Registration System (GSRS) | |
URL | https://gsrs.ncats.nih.gov/ginas/app/beta/substances/52Y8L60CR7 | |
Description | The FDA Global Substance Registration System (GSRS) enables the efficient and accurate exchange of information on what substances are in regulated products. Instead of relying on names, which vary across regulatory domains, countries, and regions, the GSRS knowledge base makes it possible for substances to be defined by standardized, scientific descriptions. | |
Explanation | Unless otherwise noted, the contents of the FDA website (www.fda.gov), both text and graphics, are not copyrighted. They are in the public domain and may be republished, reprinted and otherwise used freely by anyone without the need to obtain permission from FDA. Credit to the U.S. Food and Drug Administration as the source is appreciated but not required. | |
Disclaimer and Information on In-Vitro Research Products
Please be aware that all articles and product information presented on BenchChem are intended solely for informational purposes. The products available for purchase on BenchChem are specifically designed for in-vitro studies, which are conducted outside of living organisms. In-vitro studies, derived from the Latin term "in glass," involve experiments performed in controlled laboratory settings using cells or tissues. It is important to note that these products are not categorized as medicines or drugs, and they have not received approval from the FDA for the prevention, treatment, or cure of any medical condition, ailment, or disease. We must emphasize that any form of bodily introduction of these products into humans or animals is strictly prohibited by law. It is essential to adhere to these guidelines to ensure compliance with legal and ethical standards in research and experimentation.