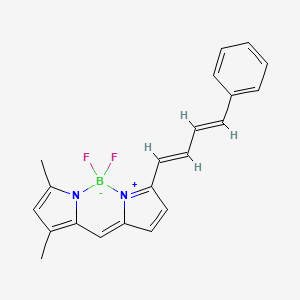
Pbd-bodipy
Overview
Description
PBD-BODIPY is a fluorescent dye used for spectrophotometric measurements of autoxidation reactions. Its chemical structure consists of a BODIPY core (boron-dipyrromethene) functionalized with a phenylboronic acid moiety. The compound’s CAS number is 148185-52-8 .
Mechanism of Action
Target of Action
Pbd-bodipy is a probe used for the spectrophotometric measurement of autoxidation reactions . It has been used to measure the activity of radical-trapping antioxidants in cell-free assays . It has also been used as a fluorescent probe for the detection of epoxidation activity .
Mode of Action
This compound serves as the signal carrier in autoxidation reactions . It enables the determination of rate constants and stoichiometries for reactions of inhibitors with chain-carrying peroxyl radicals . The co-autoxidation of the this compound signal carrier and a hydrocarbon co-substrate can be quantified by monitoring the loss of absorbance at 591 nm .
Biochemical Pathways
It is known that this compound derivatives have been used as probes in applications like imaging and sensing due to their unique properties . They have also been employed in areas like photodynamic therapy .
Pharmacokinetics
It is known that this compound is a probe for the spectrophotometric measurement of autoxidation reactions . More research is needed to understand the ADME properties of this compound and their impact on bioavailability.
Result of Action
The result of this compound’s action is the measurement of the activity of radical-trapping antioxidants in cell-free assays . It has also been used as a fluorescent probe for the detection of epoxidation activity . In addition, this compound has been used in various in vitro studies for labeling in evaluating drugs’ efficacy, localization, distribution, detection, and mechanism of action .
Action Environment
The action of this compound can be influenced by various environmental factors. For instance, BODIPY-based photocages that absorb visible/near-infrared (Vis/NIR) light offer advantages such as deeper tissue penetration and reduced bio-autofluorescence, making them highly suitable for various biomedical applications . .
Biochemical Analysis
Biochemical Properties
Pbd-bodipy interacts with various biomolecules in biochemical reactions. It is used to measure the activity of radical-trapping antioxidants in cell-free assays . The co-autoxidation of the this compound signal carrier and a hydrocarbon co-substrate can be quantified by monitoring the loss of absorbance at 591 nm .
Molecular Mechanism
The molecular mechanism of this compound involves its interaction with biomolecules during autoxidation reactions. It serves as a signal carrier, and its absorbance at 591 nm decreases as the reaction proceeds .
Preparation Methods
Synthetic Routes:
PBD-BODIPY can be synthesized through various methods, including condensation reactions between boron-dipyrromethene precursors and phenylboronic acid derivatives. These reactions lead to the formation of the BODIPY core with the attached phenylboronic acid group.
Reaction Conditions:
The specific synthetic conditions may vary, but generally involve refluxing the reactants in suitable solvents (such as dichloromethane or toluene) under inert atmosphere. Precise reaction temperatures, stoichiometry, and purification steps are crucial for obtaining high yields.
Industrial Production:
While this compound is primarily used in research, its industrial-scale production involves similar synthetic routes, optimized for efficiency and scalability.
Chemical Reactions Analysis
PBD-BODIPY undergoes several reactions:
Oxidation: It can participate in autoxidation reactions, where it acts as a radical trap. Co-autoxidation with hydrocarbon co-substrates can be quantified by monitoring absorbance loss at 591 nm.
Epoxidation Detection: this compound serves as a fluorescent probe for detecting epoxidation activity.
Common reagents include radical initiators, oxygen, and hydrocarbon substrates. The major products depend on the specific reaction conditions.
Scientific Research Applications
PBD-BODIPY finds applications in various fields:
Chemistry: Used as a probe for studying oxidation processes and radical-trapping antioxidants.
Biology: Enables visualization of cellular processes involving oxidation.
Medicine: Investigated for potential therapeutic applications.
Industry: Employed in quality control and process monitoring.
Comparison with Similar Compounds
PBD-BODIPY’s uniqueness lies in its boron-dipyrromethene core and phenylboronic acid modification. Similar compounds include other BODIPY derivatives (e.g., BODIPY-FL, BODIPY-TMR) and related fluorescent probes.
Remember that this compound’s versatility makes it a valuable tool for researchers across disciplines
Properties
IUPAC Name |
2,2-difluoro-4,6-dimethyl-12-[(1E,3E)-4-phenylbuta-1,3-dienyl]-3-aza-1-azonia-2-boranuidatricyclo[7.3.0.03,7]dodeca-1(12),4,6,8,10-pentaene | |
---|---|---|
Source | PubChem | |
URL | https://pubchem.ncbi.nlm.nih.gov | |
Description | Data deposited in or computed by PubChem | |
InChI |
InChI=1S/C21H19BF2N2/c1-16-14-17(2)25-21(16)15-20-13-12-19(26(20)22(25,23)24)11-7-6-10-18-8-4-3-5-9-18/h3-15H,1-2H3/b10-6+,11-7+ | |
Source | PubChem | |
URL | https://pubchem.ncbi.nlm.nih.gov | |
Description | Data deposited in or computed by PubChem | |
InChI Key |
BQSCNFXGIRMKAY-JMQWPVDRSA-N | |
Source | PubChem | |
URL | https://pubchem.ncbi.nlm.nih.gov | |
Description | Data deposited in or computed by PubChem | |
Canonical SMILES |
[B-]1(N2C(=CC(=C2C=C3[N+]1=C(C=C3)C=CC=CC4=CC=CC=C4)C)C)(F)F | |
Source | PubChem | |
URL | https://pubchem.ncbi.nlm.nih.gov | |
Description | Data deposited in or computed by PubChem | |
Isomeric SMILES |
[B-]1(N2C(=CC(=C2C=C3[N+]1=C(C=C3)/C=C/C=C/C4=CC=CC=C4)C)C)(F)F | |
Source | PubChem | |
URL | https://pubchem.ncbi.nlm.nih.gov | |
Description | Data deposited in or computed by PubChem | |
Molecular Formula |
C21H19BF2N2 | |
Source | PubChem | |
URL | https://pubchem.ncbi.nlm.nih.gov | |
Description | Data deposited in or computed by PubChem | |
Molecular Weight |
348.2 g/mol | |
Source | PubChem | |
URL | https://pubchem.ncbi.nlm.nih.gov | |
Description | Data deposited in or computed by PubChem | |
Retrosynthesis Analysis
AI-Powered Synthesis Planning: Our tool employs the Template_relevance Pistachio, Template_relevance Bkms_metabolic, Template_relevance Pistachio_ringbreaker, Template_relevance Reaxys, Template_relevance Reaxys_biocatalysis model, leveraging a vast database of chemical reactions to predict feasible synthetic routes.
One-Step Synthesis Focus: Specifically designed for one-step synthesis, it provides concise and direct routes for your target compounds, streamlining the synthesis process.
Accurate Predictions: Utilizing the extensive PISTACHIO, BKMS_METABOLIC, PISTACHIO_RINGBREAKER, REAXYS, REAXYS_BIOCATALYSIS database, our tool offers high-accuracy predictions, reflecting the latest in chemical research and data.
Strategy Settings
Precursor scoring | Relevance Heuristic |
---|---|
Min. plausibility | 0.01 |
Model | Template_relevance |
Template Set | Pistachio/Bkms_metabolic/Pistachio_ringbreaker/Reaxys/Reaxys_biocatalysis |
Top-N result to add to graph | 6 |
Feasible Synthetic Routes
Disclaimer and Information on In-Vitro Research Products
Please be aware that all articles and product information presented on BenchChem are intended solely for informational purposes. The products available for purchase on BenchChem are specifically designed for in-vitro studies, which are conducted outside of living organisms. In-vitro studies, derived from the Latin term "in glass," involve experiments performed in controlled laboratory settings using cells or tissues. It is important to note that these products are not categorized as medicines or drugs, and they have not received approval from the FDA for the prevention, treatment, or cure of any medical condition, ailment, or disease. We must emphasize that any form of bodily introduction of these products into humans or animals is strictly prohibited by law. It is essential to adhere to these guidelines to ensure compliance with legal and ethical standards in research and experimentation.