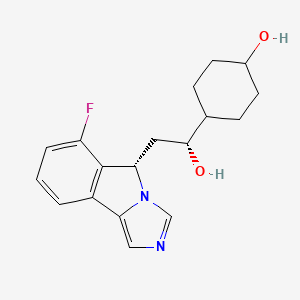
Navoximod
Overview
Description
Navoximod (GDC-0919, NLG-919) is a potent small-molecule inhibitor of indoleamine 2,3-dioxygenase 1 (IDO1), an enzyme implicated in immune tolerance within the tumor microenvironment. Preclinically, this compound exhibits an IC₅₀ of 75–90 nM in cell-based assays and enhances antitumor T-cell activity when combined with anti-PD-L1 therapies . Clinically, it demonstrates linear pharmacokinetics (PK) with dose-proportional exposure (50–1000 mg), a half-life of ~12 hours, and moderate absolute bioavailability (55.5%) . Its primary clearance mechanism is glucuronidation, forming the major metabolite M28, which accounts for 57.5% of drug-related exposure .
Preparation Methods
Stereoselective Synthesis via Crystallization-Induced Dynamic Resolution
Key Transformations and Reagents
The synthesis features two pivotal ketone reductions:
-
Biocatalytic Ketoreductase-Mediated Reduction : A ketoreductase enzyme selectively reduces a proximal ketone to install the first stereocenter with >99% ee. This step leverages the enzyme’s inherent chiral recognition, avoiding the need for protective groups .
-
Substrate-Controlled Hydride Delivery : LiAlH(Ot-Bu)₃ delivers a hydride to a second ketone, where the existing stereochemistry dictates the trajectory of reduction, ensuring correct configuration at the adjacent carbon .
Crystallization-induced dynamic resolution resolves a racemic intermediate early in the sequence, enhancing the enantiopurity of subsequent steps. This technique exploits differential solubility of diastereomers, enabling isolation of the desired isomer in >99% ee after recrystallization .
Biocatalytic and Chemoenzymatic Approaches
Role of Ketoreductases in Stereocontrol
Biocatalysis has emerged as a cornerstone of this compound synthesis, particularly in achieving high enantioselectivity. Ketoreductases (KREDs) catalyze the reduction of ketones to secondary alcohols with precise stereochemical outcomes. In one study, KRED-P1-G03 was identified as the optimal enzyme, converting the ketone intermediate to the (S)-alcohol with 99.7% ee and a turnover number (TON) of 5,000 . This step eliminates the need for chiral auxiliaries or tedious chromatographic separations, streamlining the synthesis.
Chemoenzymatic Optimization
Recent efforts have focused on integrating enzymatic and chemical steps to enhance scalability. For example, the KRED-mediated reduction is performed in a biphasic system (water:isopropyl acetate), allowing facile recovery of the product and enzyme reuse. Coupling this with flow chemistry reduced reaction times from 24 hours to 4 hours, demonstrating the potential for industrial-scale production .
Large-Scale Preparation and Formulation Considerations
Radiolabelled Synthesis for Pharmacokinetic Studies
To support clinical trials, radiolabelled [¹⁴C]-navoximod was synthesized for absolute bioavailability studies. The intravenous (IV) formulation contained 5 mg/mL this compound in 0.9% sodium chloride, while the oral solution used 200 mg/mL [¹⁴C]-navoximod in water. Both formulations maintained >98% chemical stability over 24 hours at room temperature, critical for ensuring accurate pharmacokinetic data .
Solubility and Stability Profiling
This compound exhibits high aqueous solubility (200 mg/mL), facilitating formulation as a clear, colorless solution. Stability studies under accelerated conditions (40°C, 75% humidity) confirmed no degradation over 12 months, with impurities remaining below 0.5% as measured by high-performance liquid chromatography (HPLC) .
Comparative Analysis of Synthetic Routes
Efficiency Metrics Across Methodologies
The table below contrasts the efficiency of the primary synthetic route with alternative approaches:
While the hydroxyindazole route offers fewer steps, its lower yield and lack of stereochemical data limit utility for this compound production .
Cost and Scalability
The biocatalytic steps in the 10-step route reduce reliance on expensive chiral catalysts, cutting raw material costs by ~40% compared to traditional asymmetric hydrogenation. However, the requirement for cryogenic conditions (−20°C) during LiAlH(Ot-Bu)₃-mediated reductions introduces energy costs, necessitating trade-offs in process design .
Chemical Reactions Analysis
Navoximod undergoes various chemical reactions, including:
Oxidation: this compound can be oxidized under specific conditions to form different oxidation products.
Reduction: Reduction reactions can be used to modify the functional groups in this compound.
Substitution: this compound can undergo substitution reactions where functional groups are replaced by other groups.
Common reagents used in these reactions include oxidizing agents, reducing agents, and various catalysts. The major products formed from these reactions depend on the specific conditions and reagents used .
Scientific Research Applications
Navoximod has a wide range of scientific research applications, particularly in the fields of chemistry, biology, medicine, and industry:
Chemistry: this compound is used as a model compound to study the synthesis and reactions of IDO1 inhibitors.
Biology: In biological research, this compound is used to investigate the role of IDO1 in immune regulation and tumor biology.
Medicine: this compound is being explored as a potential therapeutic agent in cancer immunotherapy. .
Industry: In the pharmaceutical industry, this compound is being developed as a drug candidate for cancer treatment.
Mechanism of Action
Navoximod exerts its effects by inhibiting the activity of IDO1, an enzyme involved in the catabolism of L-tryptophan to kynurenine . By blocking this pathway, this compound reduces the accumulation of kynurenine metabolites, which are known to suppress T-cell function and promote tumor immune escape . The inhibition of IDO1 by this compound leads to the restoration of T-cell activity and enhances the immune system’s ability to detect and eliminate tumor cells .
Comparison with Similar Compounds
Target Selectivity and Mechanism
Key Findings :
- This compound’s dual inhibition of IDO1 and TDO may broaden therapeutic impact but risks off-target effects, whereas epacadostat and BMS-986205 exhibit higher specificity .
- Indoximod lacks direct enzymatic inhibition, relying on downstream immune modulation .
Pharmacokinetic and Metabolic Profiles
Key Findings :
- This compound’s moderate bioavailability and shorter half-life necessitate twice-daily dosing, whereas BMS-986205’s prolonged half-life supports once-daily use .
- Epacadostat’s higher bioavailability and longer half-life may improve compliance but increase drug-drug interaction risks via CYP3A4 .
Key Findings :
- Epacadostat and BMS-986205 show higher response rates in combinations but face safety challenges (e.g., ECHO-301 trial failure) .
- This compound’s safety profile is tolerable, with chromaturia (55%) and rash as notable but non-life-threatening effects .
Pharmacodynamic Biomarkers
Compound | Kynurenine Reduction | Target Engagement |
---|---|---|
This compound | ~25–50% | IC₅₀ achieved at 600–1000 mg BID |
Epacadostat | ~70–90% | IC₉₀ achieved at 300 mg QD |
BMS-986205 | ~90% | Sustained target inhibition |
Key Findings :
Biological Activity
Navoximod (also known as GDC-0919) is a small-molecule inhibitor targeting indoleamine 2,3-dioxygenase 1 (IDO1) and tryptophan 2,3-dioxygenase (TDO). It has garnered attention in cancer immunotherapy due to its potential to modulate the immune response by inhibiting these enzymes, which are involved in the catabolism of tryptophan and the subsequent production of kynurenine, a metabolite that can suppress T cell activity.
This compound inhibits IDO1 and TDO, leading to decreased levels of kynurenine and increased availability of tryptophan. This shift can enhance T cell proliferation and activity, potentially improving anti-tumor immunity. The compound exhibits a dual specificity with a notable potency for IDO1, making it a candidate for combination therapies with other immunotherapeutic agents.
Pharmacokinetics and Metabolism
This compound is rapidly absorbed following oral administration, with peak plasma concentrations typically reached within one hour (T_max ~ 1 h). The half-life (t_1/2) is approximately 11 hours, supporting twice-daily dosing. It undergoes extensive metabolism primarily through glucuronidation via UDP-glucuronosyltransferases (UGT), resulting in metabolites such as M28, which accounts for a significant portion of drug exposure in humans .
Phase I Trials
Several Phase I clinical trials have evaluated the safety, tolerability, pharmacokinetics, and preliminary efficacy of this compound:
- Single-Agent Study : In an open-label study involving 22 patients with advanced cancer, this compound was administered at doses ranging from 50 to 800 mg twice daily. The study reported stable disease in 36% of patients and highlighted common adverse events such as fatigue (59%) and gastrointestinal issues .
- Combination Therapy : A subsequent trial combined this compound with atezolizumab (a PD-L1 inhibitor) in 157 patients across various tumor types. The maximum administered dose reached was 1000 mg BID without reaching the maximum tolerated dose (MTD). Notably, treatment-related adverse events included fatigue (22%) and rash (22%). Some patients experienced partial responses across multiple cancer types .
Summary of Clinical Findings
Study Type | Number of Patients | Dose Range (mg) | Common AEs (%) | Stable Disease (%) | Partial Response (%) |
---|---|---|---|---|---|
Single-Agent Phase I | 22 | 50 - 800 | Fatigue (59), Nausea (36) | 36 | - |
Combination Phase I | 157 | 50 - 1000 | Fatigue (22), Rash (22) | - | 9 |
Biological Activity Insights
The biological activity of this compound is characterized by its ability to modulate immune responses through IDO inhibition. By decreasing kynurenine levels, this compound promotes T cell activation and may counteract tumor-induced immune suppression. However, the clinical efficacy remains under investigation as results have shown mixed responses in terms of tumor regression.
Q & A
Basic Research Questions
Q. What is the molecular mechanism of Navoximod in modulating the tumor microenvironment?
this compound inhibits indoleamine 2,3-dioxygenase 1 (IDO1), an enzyme that catalyzes tryptophan (Trp) degradation into kynurenine (Kyn). This reduces Trp depletion and Kyn accumulation, reversing immunosuppression by restoring CD8+ T cell activity and suppressing regulatory T cells (Tregs). Preclinical studies show an EC50 of 70–90 nM in cellular assays and MLR assays, with synergistic effects when combined with anti-PD-L1 or chemotherapy .
Q. How are pharmacokinetic (PK) parameters of this compound assessed in clinical trials?
Plasma concentrations are quantified via validated LC/MS-MS methods (lower limit: 1 ng/mL), with non-compartmental analysis using Phoenix WinNonlin. Key PK parameters include rapid absorption (median Tmax ~1 hour), dose-proportional exposure, and a half-life (~11 hours) supporting twice-daily (BID) dosing. Stability in plasma is confirmed over multiple freeze-thaw cycles and storage conditions .
Q. What were the primary endpoints and dosing strategies in the Phase Ia trial of this compound?
The Phase Ia trial (NCT02048709) evaluated safety, maximum tolerated dose (MTD), and PK/PD in advanced solid tumors. A modified 3+3 design escalated doses from 50 mg to 800 mg BID. No MTD was reached, and the recommended Phase II dose was 600 mg BID. Pharmacodynamic effects included transient reductions in plasma Kyn, consistent with IDO1 inhibition .
Q. What preclinical models support this compound’s combination with immune checkpoint inhibitors?
In murine models (e.g., B16F10 melanoma), this compound combined with anti-PD-L1 or anti-OX40 enhanced intratumoral CD8+ T cell activation and tumor growth inhibition compared to monotherapies. This synergy is attributed to dual blockade of IDO1-mediated immunosuppression and PD-L1-driven T cell exhaustion .
Advanced Research Questions
Q. How does this compound’s dual inhibition of IDO1 and TDO influence its therapeutic profile?
this compound exhibits 10–20-fold selectivity for IDO1 over TDO (EC50: 1.5 µM for TDO). While dual inhibition may broaden efficacy in tumors co-expressing both enzymes, it raises concerns about off-target effects. Preclinical data suggest TDO inhibition contributes to plasma Kyn reduction, but clinical relevance remains unclear due to limited tissue penetration in humans .
Q. What methodological challenges arise in interpreting pharmacodynamic (PD) biomarkers like Kyn/Trp ratios?
Plasma Kyn/Trp ratios are used to assess IDO1 inhibition, but variability in tumor vs. systemic effects complicates interpretation. For example, this compound reduced plasma Kyn by ~30% in Phase Ia trials, but intratumoral Kyn modulation was not directly measured. Complementary methods (e.g., tumor biopsies, single-cell RNA sequencing) are needed to correlate PD markers with immune cell infiltration .
Q. Why did this compound fail to improve efficacy in combination with anti-PD-L1 in later-stage trials?
Despite preclinical synergy, Phase Ib/II trials combining this compound with atezolizumab showed modest activity (9% response rate) and no survival benefit. Potential factors include insufficient Kyn pathway inhibition, redundant immunosuppressive pathways, and lack of patient stratification by IDO1 expression or tumor mutational burden. This mirrors failures of other IDO1 inhibitors (e.g., epacadostat in ECHO-301) .
Q. What experimental models are suitable for studying this compound’s role in oncolytic virotherapy?
In hepatocellular carcinoma models, this compound enhanced HSV-1 replication and tumor lysis by reversing IDO1-mediated antiviral immunity. Researchers should employ syngeneic models with intact immune systems and measure viral load, cytokine profiles (e.g., IFN-γ), and immune cell recruitment to validate combination strategies .
Q. How do genetic polymorphisms (e.g., UGT1A1) affect this compound’s pharmacokinetics?
Patients with UGT1A1 *28/*28 polymorphisms showed higher this compound exposure due to reduced glucuronidation. Dose adjustments may be necessary in these populations to avoid toxicity. Pharmacogenomic analyses in Phase I trials identified UGT1A1 variants as key modifiers of PK variability .
Q. What lessons from this compound’s clinical development inform future IDO1 inhibitor trials?
Key takeaways include:
- Prioritize combination therapies with complementary mechanisms (e.g., vaccines, radiotherapy).
- Validate intratumoral PD biomarkers beyond plasma Kyn.
- Enrich trials for patients with high IDO1 expression or specific tumor subtypes (e.g., endometrial cancer).
- Address metabolic crosstalk (e.g., TDO upregulation) through dual-target inhibitors .
Q. Methodological Resources
- PK/PD Analysis : Use Phoenix WinNonlin for non-compartmental modeling of this compound’s linear PK profile .
- Biomarker Assays : Quantify Kyn/Trp via HPLC or mass spectrometry; correlate with tumor RNA-seq data for IDO1 expression .
- Preclinical Models : B16F10 melanoma for combination studies; patient-derived xenografts (PDXs) for translational validation .
Properties
IUPAC Name |
4-[(1R)-2-[(5S)-6-fluoro-5H-imidazo[5,1-a]isoindol-5-yl]-1-hydroxyethyl]cyclohexan-1-ol | |
---|---|---|
Source | PubChem | |
URL | https://pubchem.ncbi.nlm.nih.gov | |
Description | Data deposited in or computed by PubChem | |
InChI |
InChI=1S/C18H21FN2O2/c19-14-3-1-2-13-16-9-20-10-21(16)15(18(13)14)8-17(23)11-4-6-12(22)7-5-11/h1-3,9-12,15,17,22-23H,4-8H2/t11?,12?,15-,17+/m0/s1 | |
Source | PubChem | |
URL | https://pubchem.ncbi.nlm.nih.gov | |
Description | Data deposited in or computed by PubChem | |
InChI Key |
YGACXVRLDHEXKY-LHPNLFKDSA-N | |
Source | PubChem | |
URL | https://pubchem.ncbi.nlm.nih.gov | |
Description | Data deposited in or computed by PubChem | |
Canonical SMILES |
C1CC(CCC1C(CC2C3=C(C=CC=C3F)C4=CN=CN24)O)O | |
Source | PubChem | |
URL | https://pubchem.ncbi.nlm.nih.gov | |
Description | Data deposited in or computed by PubChem | |
Isomeric SMILES |
C1CC(CCC1[C@@H](C[C@H]2C3=C(C=CC=C3F)C4=CN=CN24)O)O | |
Source | PubChem | |
URL | https://pubchem.ncbi.nlm.nih.gov | |
Description | Data deposited in or computed by PubChem | |
Molecular Formula |
C18H21FN2O2 | |
Source | PubChem | |
URL | https://pubchem.ncbi.nlm.nih.gov | |
Description | Data deposited in or computed by PubChem | |
Molecular Weight |
316.4 g/mol | |
Source | PubChem | |
URL | https://pubchem.ncbi.nlm.nih.gov | |
Description | Data deposited in or computed by PubChem | |
CAS No. |
1402837-78-8 | |
Record name | Navoximod [USAN] | |
Source | ChemIDplus | |
URL | https://pubchem.ncbi.nlm.nih.gov/substance/?source=chemidplus&sourceid=1402837788 | |
Description | ChemIDplus is a free, web search system that provides access to the structure and nomenclature authority files used for the identification of chemical substances cited in National Library of Medicine (NLM) databases, including the TOXNET system. | |
Record name | Navoximod | |
Source | DrugBank | |
URL | https://www.drugbank.ca/drugs/DB15439 | |
Description | The DrugBank database is a unique bioinformatics and cheminformatics resource that combines detailed drug (i.e. chemical, pharmacological and pharmaceutical) data with comprehensive drug target (i.e. sequence, structure, and pathway) information. | |
Explanation | Creative Common's Attribution-NonCommercial 4.0 International License (http://creativecommons.org/licenses/by-nc/4.0/legalcode) | |
Record name | NAVOXIMOD | |
Source | FDA Global Substance Registration System (GSRS) | |
URL | https://gsrs.ncats.nih.gov/ginas/app/beta/substances/926SHL95NC | |
Description | The FDA Global Substance Registration System (GSRS) enables the efficient and accurate exchange of information on what substances are in regulated products. Instead of relying on names, which vary across regulatory domains, countries, and regions, the GSRS knowledge base makes it possible for substances to be defined by standardized, scientific descriptions. | |
Explanation | Unless otherwise noted, the contents of the FDA website (www.fda.gov), both text and graphics, are not copyrighted. They are in the public domain and may be republished, reprinted and otherwise used freely by anyone without the need to obtain permission from FDA. Credit to the U.S. Food and Drug Administration as the source is appreciated but not required. | |
Disclaimer and Information on In-Vitro Research Products
Please be aware that all articles and product information presented on BenchChem are intended solely for informational purposes. The products available for purchase on BenchChem are specifically designed for in-vitro studies, which are conducted outside of living organisms. In-vitro studies, derived from the Latin term "in glass," involve experiments performed in controlled laboratory settings using cells or tissues. It is important to note that these products are not categorized as medicines or drugs, and they have not received approval from the FDA for the prevention, treatment, or cure of any medical condition, ailment, or disease. We must emphasize that any form of bodily introduction of these products into humans or animals is strictly prohibited by law. It is essential to adhere to these guidelines to ensure compliance with legal and ethical standards in research and experimentation.