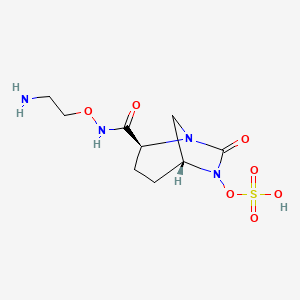
Nacubactam
Overview
Description
Nacubactam is a novel β-lactamase inhibitor . It has dual mechanisms of action as an inhibitor of serine β-lactamases (classes A and C and some class D) and an inhibitor of penicillin binding protein 2 in Enterobacterales .
Synthesis Analysis
Nacubactam is a bridged diazabicyclooctane β-lactamase inhibitor that inactivates class A and class C β-lactamases . It functions both as an independent antibiotic as well as providing “enhancement” when combined with β-lactam antibiotics with potent activity against Enterobacterales .
Molecular Structure Analysis
The molecular formula of Nacubactam is C9H16N4O7S . The IUPAC name is [(2S,5R)-2-(2-aminoethoxycarbamoyl)-7-oxo-1,6-diazabicyclo[3.2.1]octan-6-yl] hydrogen sulfate . The molecular weight is 324.31 g/mol .
Chemical Reactions Analysis
Nacubactam has been evaluated in single- and multiple-ascending-dose, placebo-controlled studies . Healthy participants received single ascending doses of nacubactam of 50 to 8,000 mg, multiple ascending doses of nacubactam of 1,000 to 4,000 mg every 8 h (q8h) for up to 7 days, or nacubactam of 2,000 mg plus meropenem of 2,000 mg q8h for 6 days after a 3-day lead-in period .
Physical And Chemical Properties Analysis
Nacubactam is a compound with the CID 73386748 in PubChem . The InChI is 1S/C9H16N4O7S/c10-3-4-19-11-8(14)7-2-1-6-5-12(7)9(15)13(6)20-21(16,17)18/h6-7H,1-5,10H2,(H,11,14)(H,16,17,18)/t6-,7+/m1/s1 . The Canonical SMILES is C1CC(N2CC1N(C2=O)OS(=O)(=O)O)C(=O)NOCCN .
Scientific Research Applications
Treatment of Carbapenem-Resistant Infections
Nacubactam has shown promise in treating infections caused by Carbapenem-Resistant Enterobacteriaceae (CRE) . It enhances the efficacy of β-lactams like aztreonam, cefepime, and meropenem against CRE and carbapenemase-producing strains such as Enterobacter cloacae and Klebsiella pneumoniae .
Inhibition of β-Lactamase Enzymes
As a β-lactamase inhibitor, Nacubactam targets enzymes that confer resistance to β-lactam antibiotics. It’s particularly effective against class A and class C β-lactamases, expanding the utility of β-lactam antibiotics against resistant bacterial strains .
Enhancing Antibiotic Potency
Combination therapies involving Nacubactam have been shown to inhibit a significant percentage of metallo-β-lactamase (MBL) producing Enterobacterales. This includes enhancing the potency of drugs like meropenem and cefepime, which are critical in the fight against multidrug-resistant pathogens .
Addressing Antimicrobial Resistance
Nacubactam is part of a new wave of carbapenemase inhibitors that aim to tackle the global health problem of antimicrobial resistance. Its development represents a significant step forward in restoring the effectiveness of β-lactam antibiotics against resistant organisms .
Pneumonia Treatment in Animal Models
In murine pneumonia models, Nacubactam has demonstrated enhanced antimicrobial activity when used in combination with β-lactams. This suggests potential applications in treating pneumonia caused by resistant bacterial strains in humans .
Potential for Clinical Use
While still in the development stages, Nacubactam has undergone preclinical trials, showing promising results that pave the way for its use in clinical settings. Its ability to inhibit a broad range of β-lactamase enzymes makes it a valuable candidate for future therapeutic applications .
Mechanism of Action
Target of Action
Nacubactam, also known as OP-0595, primarily targets β-lactamase enzymes and penicillin-binding protein 2 . β-lactamase enzymes are responsible for the emergence of antibiotic resistance, particularly in Gram-negative bacteria . Penicillin-binding protein 2 plays a crucial role in bacterial cell wall synthesis .
Mode of Action
Nacubactam exhibits a dual mechanism of action. It inhibits serine β-lactamases (classes A, C, and some class D) and penicillin-binding protein 2 . By inhibiting these targets, Nacubactam prevents the hydrolysis of co-administered β-lactam antibiotics, thereby enhancing their antibacterial activity .
Biochemical Pathways
Nacubactam affects the biochemical pathways associated with bacterial resistance to β-lactam antibiotics. By inhibiting β-lactamase enzymes and penicillin-binding protein 2, Nacubactam disrupts the bacterial cell wall synthesis pathway, leading to the death of the bacteria .
Pharmacokinetics
The pharmacokinetic properties of Nacubactam are still under investigation . It is known that nacubactam is largely excreted unchanged into urine . The pharmacokinetics of Nacubactam appear linear, and exposure increases in an approximately dose-proportional manner across the dose range investigated .
Result of Action
The inhibition of β-lactamase enzymes and penicillin-binding protein 2 by Nacubactam results in the prevention of β-lactam antibiotic hydrolysis, thereby enhancing the antibacterial activity of these antibiotics . This leads to the death of the bacteria, effectively combating antibiotic resistance .
Action Environment
The efficacy and stability of Nacubactam can be influenced by various environmental factors. For instance, changes in the permeability of the bacterial cell membrane and the intrinsic activity of efflux pumps can limit drug absorption and render antibiotics ineffective . .
properties
IUPAC Name |
[(2S,5R)-2-(2-aminoethoxycarbamoyl)-7-oxo-1,6-diazabicyclo[3.2.1]octan-6-yl] hydrogen sulfate | |
---|---|---|
Source | PubChem | |
URL | https://pubchem.ncbi.nlm.nih.gov | |
Description | Data deposited in or computed by PubChem | |
InChI |
InChI=1S/C9H16N4O7S/c10-3-4-19-11-8(14)7-2-1-6-5-12(7)9(15)13(6)20-21(16,17)18/h6-7H,1-5,10H2,(H,11,14)(H,16,17,18)/t6-,7+/m1/s1 | |
Source | PubChem | |
URL | https://pubchem.ncbi.nlm.nih.gov | |
Description | Data deposited in or computed by PubChem | |
InChI Key |
RSBPYSTVZQAADE-RQJHMYQMSA-N | |
Source | PubChem | |
URL | https://pubchem.ncbi.nlm.nih.gov | |
Description | Data deposited in or computed by PubChem | |
Canonical SMILES |
C1CC(N2CC1N(C2=O)OS(=O)(=O)O)C(=O)NOCCN | |
Source | PubChem | |
URL | https://pubchem.ncbi.nlm.nih.gov | |
Description | Data deposited in or computed by PubChem | |
Isomeric SMILES |
C1C[C@H](N2C[C@@H]1N(C2=O)OS(=O)(=O)O)C(=O)NOCCN | |
Source | PubChem | |
URL | https://pubchem.ncbi.nlm.nih.gov | |
Description | Data deposited in or computed by PubChem | |
Molecular Formula |
C9H16N4O7S | |
Source | PubChem | |
URL | https://pubchem.ncbi.nlm.nih.gov | |
Description | Data deposited in or computed by PubChem | |
Molecular Weight |
324.31 g/mol | |
Source | PubChem | |
URL | https://pubchem.ncbi.nlm.nih.gov | |
Description | Data deposited in or computed by PubChem | |
Product Name |
Nacubactam | |
CAS RN |
1452458-86-4 | |
Record name | Nacubactam [USAN:INN] | |
Source | ChemIDplus | |
URL | https://pubchem.ncbi.nlm.nih.gov/substance/?source=chemidplus&sourceid=1452458864 | |
Description | ChemIDplus is a free, web search system that provides access to the structure and nomenclature authority files used for the identification of chemical substances cited in National Library of Medicine (NLM) databases, including the TOXNET system. | |
Record name | Nacubactam | |
Source | DrugBank | |
URL | https://www.drugbank.ca/drugs/DB15353 | |
Description | The DrugBank database is a unique bioinformatics and cheminformatics resource that combines detailed drug (i.e. chemical, pharmacological and pharmaceutical) data with comprehensive drug target (i.e. sequence, structure, and pathway) information. | |
Explanation | Creative Common's Attribution-NonCommercial 4.0 International License (http://creativecommons.org/licenses/by-nc/4.0/legalcode) | |
Record name | (2S,5R)-N-(aminoethoxy)-7-oxo-6-(sulfooxy)-1,6-diazobicylo[3.2.1]octane-2-carboxamide | |
Source | European Chemicals Agency (ECHA) | |
URL | https://echa.europa.eu/information-on-chemicals | |
Description | The European Chemicals Agency (ECHA) is an agency of the European Union which is the driving force among regulatory authorities in implementing the EU's groundbreaking chemicals legislation for the benefit of human health and the environment as well as for innovation and competitiveness. | |
Explanation | Use of the information, documents and data from the ECHA website is subject to the terms and conditions of this Legal Notice, and subject to other binding limitations provided for under applicable law, the information, documents and data made available on the ECHA website may be reproduced, distributed and/or used, totally or in part, for non-commercial purposes provided that ECHA is acknowledged as the source: "Source: European Chemicals Agency, http://echa.europa.eu/". Such acknowledgement must be included in each copy of the material. ECHA permits and encourages organisations and individuals to create links to the ECHA website under the following cumulative conditions: Links can only be made to webpages that provide a link to the Legal Notice page. | |
Record name | NACUBACTAM | |
Source | FDA Global Substance Registration System (GSRS) | |
URL | https://gsrs.ncats.nih.gov/ginas/app/beta/substances/832O37V7MZ | |
Description | The FDA Global Substance Registration System (GSRS) enables the efficient and accurate exchange of information on what substances are in regulated products. Instead of relying on names, which vary across regulatory domains, countries, and regions, the GSRS knowledge base makes it possible for substances to be defined by standardized, scientific descriptions. | |
Explanation | Unless otherwise noted, the contents of the FDA website (www.fda.gov), both text and graphics, are not copyrighted. They are in the public domain and may be republished, reprinted and otherwise used freely by anyone without the need to obtain permission from FDA. Credit to the U.S. Food and Drug Administration as the source is appreciated but not required. | |
Disclaimer and Information on In-Vitro Research Products
Please be aware that all articles and product information presented on BenchChem are intended solely for informational purposes. The products available for purchase on BenchChem are specifically designed for in-vitro studies, which are conducted outside of living organisms. In-vitro studies, derived from the Latin term "in glass," involve experiments performed in controlled laboratory settings using cells or tissues. It is important to note that these products are not categorized as medicines or drugs, and they have not received approval from the FDA for the prevention, treatment, or cure of any medical condition, ailment, or disease. We must emphasize that any form of bodily introduction of these products into humans or animals is strictly prohibited by law. It is essential to adhere to these guidelines to ensure compliance with legal and ethical standards in research and experimentation.