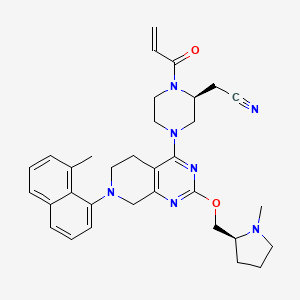
MRTX-1257
Overview
Description
MRTX-1257 is a selective, covalent inhibitor of the KRAS G12C mutation, which is a common oncogenic driver in various cancers, including non-small cell lung cancer and colorectal cancer . This compound has shown promising results in preclinical studies, demonstrating its potential as a therapeutic agent for targeting KRAS G12C-mutated cancers .
Mechanism of Action
Target of Action
MRTX-1257 is a selective, irreversible, covalent inhibitor of KRAS G12C . KRAS G12C is an established driver mutation in various cancers . This mutation has been historically challenging to target, but recent advancements have led to the development of selective inhibitors like this compound .
Mode of Action
This compound interacts with its target, KRAS G12C, through a covalent bond . This interaction is facilitated by the close proximity of Cysteine 12 to both the nucleotide pocket and the switch regions of the KRAS protein . The compound’s thiol-reactive property allows it to bind to the active site of KRAS G12C and inhibit its mutation-driven signaling .
Biochemical Pathways
The primary biochemical pathway affected by this compound is the RAS signaling pathway . By inhibiting KRAS G12C, this compound suppresses downstream effector signaling, particularly the ERK phosphorylation pathway . This leads to a reduction in cell viability and tumor growth .
Pharmacokinetics
This compound has demonstrated favorable oral properties and low nanomolar cell potency . It has an IC50 of 900 pM for KRAS-dependent ERK phosphorylation in H358 cells . In animal models, this compound demonstrated 31% bioavailability, with free fraction exposures well above the cellular potency .
Result of Action
The molecular and cellular effects of this compound’s action include potent reduction in effector signaling in cells with the G12C mutation . This leads to a decrease in cell viability and tumor growth . In animal models, this compound showed rapid tumor growth inhibition at all tested concentrations .
Action Environment
The efficacy and stability of this compound can be influenced by various environmental factors. For instance, the compound was found to be more potent in 3D cell culture conditions compared to 2D . Additionally, there was heterogeneity in the occurrence of additivity/synergy amongst the KRAS G12C mutant lines in combination studies, indicating that factors in addition to KRAS mutations influenced response .
Biochemical Analysis
Biochemical Properties
MRTX-1257 plays a crucial role in biochemical reactions by selectively inhibiting the KRAS G12C protein. This inhibition is achieved through a covalent bond formation with the cysteine residue at codon 12 of KRAS, which stabilizes the protein in its inactive GDP-bound state . The compound has an IC50 of 900 pM for KRAS-dependent ERK phosphorylation in H358 cells . This compound interacts with various biomolecules, including enzymes and proteins involved in the KRAS signaling pathway, thereby disrupting downstream signaling events essential for cancer cell proliferation .
Cellular Effects
This compound exerts significant effects on various cell types, particularly those harboring the KRAS G12C mutation. In cellular models, this compound inhibits cell proliferation and induces apoptosis in KRAS G12C-mutant cells . The compound influences cell signaling pathways by inhibiting ERK phosphorylation, which is a critical downstream effector of KRAS . Additionally, this compound affects gene expression and cellular metabolism by altering the activity of key signaling molecules involved in cell growth and survival .
Molecular Mechanism
The molecular mechanism of this compound involves its selective binding to the KRAS G12C protein. By forming a covalent bond with the cysteine residue at codon 12, this compound locks KRAS in its inactive GDP-bound state . This binding prevents the activation of downstream signaling pathways, such as the MAPK/ERK pathway, which are essential for cell proliferation and survival . The compound’s high selectivity for KRAS G12C over other KRAS isoforms and its ability to irreversibly inhibit the protein make it a potent therapeutic agent .
Temporal Effects in Laboratory Settings
In laboratory settings, the effects of this compound have been observed to change over time. The compound demonstrates rapid and sustained inhibition of tumor growth in in vivo models . This compound is stable under various storage conditions, with a shelf life of several months when stored at -20°C . Long-term studies have shown that the compound maintains its efficacy in inhibiting KRAS signaling and tumor growth over extended periods .
Dosage Effects in Animal Models
The effects of this compound vary with different dosages in animal models. In studies involving mice, this compound has been administered at doses ranging from 1 mg/kg to 100 mg/kg . The compound shows rapid tumor growth inhibition at all dose levels, with sustained regression observed at higher doses (3 mg/kg, 10 mg/kg, 30 mg/kg, and 100 mg/kg) . At the highest dose of 100 mg/kg, this compound leads to complete tumor regression, which is maintained for over 70 days after treatment cessation . Higher doses may also result in toxic or adverse effects .
Metabolic Pathways
This compound is involved in metabolic pathways related to its inhibition of KRAS G12C. The compound interacts with enzymes and cofactors that regulate KRAS signaling and cellular metabolism . By inhibiting KRAS, this compound disrupts metabolic flux and alters metabolite levels, leading to reduced cell proliferation and increased apoptosis in cancer cells .
Transport and Distribution
Within cells and tissues, this compound is transported and distributed through various mechanisms. The compound exhibits medium permeability and efflux, with high hepatocyte clearance . This compound is orally active and demonstrates good bioavailability in animal models, with a bioavailability of 31% at a 30 mg/kg dose . The compound’s distribution is influenced by its interactions with transporters and binding proteins that facilitate its localization to target tissues .
Subcellular Localization
This compound’s subcellular localization is primarily within the cytoplasm, where it interacts with KRAS G12C . The compound’s targeting signals and post-translational modifications direct it to specific compartments, ensuring its effective inhibition of KRAS signaling . This localization is critical for the compound’s activity and function, as it allows this compound to effectively bind and inhibit its target protein .
Preparation Methods
The synthesis of MRTX-1257 involves structure-based drug design, utilizing substituted tetrahydropyridopyrimidines as the core structure . The key steps in the synthetic route include:
Formation of the tetrahydropyridopyrimidine core: This involves the cyclization of appropriate precursors under controlled conditions.
Introduction of functional groups: The cyanomethyl group and the 8-methylnaphthyl group are introduced to enhance the potency and selectivity of the compound.
Optimization of molecular interactions: Iterative structure-based optimization is performed to fine-tune the interactions with the KRAS G12C protein.
Industrial production methods for this compound are not extensively documented, but they likely involve large-scale synthesis following the optimized synthetic route established in preclinical studies.
Chemical Reactions Analysis
MRTX-1257 undergoes several key chemical reactions:
Covalent Bond Formation: This compound forms a covalent bond with the cysteine residue at codon 12 of the KRAS G12C protein.
Binding in the Switch-2 Pocket: The compound binds in the “Switch-2” pocket of KRAS, stabilizing the protein in its inactive, GDP-bound state.
Displacement of Water Molecule: The cyanomethyl group displaces a water molecule found near Gly10 in co-crystal structures of less potent analogs.
Common reagents and conditions used in these reactions include organic solvents, controlled temperatures, and specific catalysts to facilitate the formation of the desired covalent bonds and molecular interactions.
Scientific Research Applications
MRTX-1257 has several scientific research applications:
Cancer Research: It is used as a research tool to study the therapeutic susceptibility and dependence of KRAS-mutated cancers.
Drug Development: This compound serves as a lead compound for developing new inhibitors targeting KRAS G12C mutations.
Combination Therapy Studies: It is evaluated in combination with other anticancer agents to explore synergistic effects and improve therapeutic outcomes.
Comparison with Similar Compounds
MRTX-1257 is compared with other KRAS G12C inhibitors, such as sotorasib (AMG-510) and TNO-155 . While all these compounds target the same mutation, this compound is unique in its structure and binding interactions:
Sotorasib (AMG-510): Another selective KRAS G12C inhibitor with a different chemical structure and binding profile.
This compound stands out due to its specific molecular interactions and enhanced potency compared to unsubstituted analogs .
Properties
IUPAC Name |
2-[(2S)-4-[7-(8-methylnaphthalen-1-yl)-2-[[(2S)-1-methylpyrrolidin-2-yl]methoxy]-6,8-dihydro-5H-pyrido[3,4-d]pyrimidin-4-yl]-1-prop-2-enoylpiperazin-2-yl]acetonitrile | |
---|---|---|
Source | PubChem | |
URL | https://pubchem.ncbi.nlm.nih.gov | |
Description | Data deposited in or computed by PubChem | |
InChI |
InChI=1S/C33H39N7O2/c1-4-30(41)40-19-18-39(20-25(40)13-15-34)32-27-14-17-38(29-12-6-10-24-9-5-8-23(2)31(24)29)21-28(27)35-33(36-32)42-22-26-11-7-16-37(26)3/h4-6,8-10,12,25-26H,1,7,11,13-14,16-22H2,2-3H3/t25-,26-/m0/s1 | |
Source | PubChem | |
URL | https://pubchem.ncbi.nlm.nih.gov | |
Description | Data deposited in or computed by PubChem | |
InChI Key |
YRYQLVCTQFBRLD-UIOOFZCWSA-N | |
Source | PubChem | |
URL | https://pubchem.ncbi.nlm.nih.gov | |
Description | Data deposited in or computed by PubChem | |
Canonical SMILES |
CC1=C2C(=CC=C1)C=CC=C2N3CCC4=C(C3)N=C(N=C4N5CCN(C(C5)CC#N)C(=O)C=C)OCC6CCCN6C | |
Source | PubChem | |
URL | https://pubchem.ncbi.nlm.nih.gov | |
Description | Data deposited in or computed by PubChem | |
Isomeric SMILES |
CC1=C2C(=CC=C1)C=CC=C2N3CCC4=C(C3)N=C(N=C4N5CCN([C@H](C5)CC#N)C(=O)C=C)OC[C@@H]6CCCN6C | |
Source | PubChem | |
URL | https://pubchem.ncbi.nlm.nih.gov | |
Description | Data deposited in or computed by PubChem | |
Molecular Formula |
C33H39N7O2 | |
Source | PubChem | |
URL | https://pubchem.ncbi.nlm.nih.gov | |
Description | Data deposited in or computed by PubChem | |
Molecular Weight |
565.7 g/mol | |
Source | PubChem | |
URL | https://pubchem.ncbi.nlm.nih.gov | |
Description | Data deposited in or computed by PubChem | |
Retrosynthesis Analysis
AI-Powered Synthesis Planning: Our tool employs the Template_relevance Pistachio, Template_relevance Bkms_metabolic, Template_relevance Pistachio_ringbreaker, Template_relevance Reaxys, Template_relevance Reaxys_biocatalysis model, leveraging a vast database of chemical reactions to predict feasible synthetic routes.
One-Step Synthesis Focus: Specifically designed for one-step synthesis, it provides concise and direct routes for your target compounds, streamlining the synthesis process.
Accurate Predictions: Utilizing the extensive PISTACHIO, BKMS_METABOLIC, PISTACHIO_RINGBREAKER, REAXYS, REAXYS_BIOCATALYSIS database, our tool offers high-accuracy predictions, reflecting the latest in chemical research and data.
Strategy Settings
Precursor scoring | Relevance Heuristic |
---|---|
Min. plausibility | 0.01 |
Model | Template_relevance |
Template Set | Pistachio/Bkms_metabolic/Pistachio_ringbreaker/Reaxys/Reaxys_biocatalysis |
Top-N result to add to graph | 6 |
Feasible Synthetic Routes
Disclaimer and Information on In-Vitro Research Products
Please be aware that all articles and product information presented on BenchChem are intended solely for informational purposes. The products available for purchase on BenchChem are specifically designed for in-vitro studies, which are conducted outside of living organisms. In-vitro studies, derived from the Latin term "in glass," involve experiments performed in controlled laboratory settings using cells or tissues. It is important to note that these products are not categorized as medicines or drugs, and they have not received approval from the FDA for the prevention, treatment, or cure of any medical condition, ailment, or disease. We must emphasize that any form of bodily introduction of these products into humans or animals is strictly prohibited by law. It is essential to adhere to these guidelines to ensure compliance with legal and ethical standards in research and experimentation.