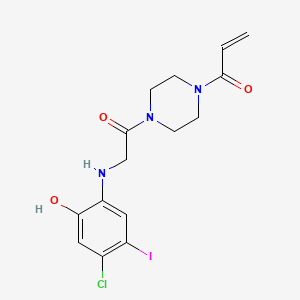
K-Ras(G12C) inhibitor 12
- Click on QUICK INQUIRY to receive a quote from our team of experts.
- With the quality product at a COMPETITIVE price, you can focus more on your research.
Overview
Description
K-Ras(G12C) inhibitor 12 irreversibly binds to the oncogenic mutant K-Ras(G12C), blocking K-Ras(G12C) interactions. K-Ras is a small GTPase that cycles between a GTP-bound active state and a GDP-bound inactive state to turn on downstream Raf kinases to drive cell growth. A G12C mutation in K-Ras blocks GTP hydrolysis, activates K-Ras, and promotes carcinogenesis. This compound has been used to decrease viability and increase apoptosis in several lung cancer cell lines containing G12C mutations (EC50 = 0.32 µM in H1792 cells).
Mechanism of Action
Target of Action
The primary target of K-Ras(G12C) inhibitor 12 is the K-Ras protein, specifically the G12C mutant variant . K-Ras is a GTPase and plays an important role in many signal transduction pathways . The mutation of K-Ras occurs in many cancers .
Mode of Action
this compound is an allosteric inhibitor . It binds to the K-Ras(G12C) protein, significantly reducing its affinity for GTP and leading to the modification of K-Ras(G12C) . This binding inhibits the activation of the receptor and the subsequent signal-transduction pathway .
Biochemical Pathways
The inhibitor preferentially binds RAS in the GDP-bound conformation, blocking the exchange with GTP and thus preventing the activation of the signaling cascade . This results in the inhibition of multiple downstream pathways including the RAF–MEK–MAPK and PI3K–AKT–mTOR pathways which stimulate cell growth and survival .
Pharmacokinetics
It’s known that the inhibitor rapidly binds to kras g12c, increasing the reaction rate in a biochemical assay by 600-fold . It also blocks nucleotide exchange (GDP to GTP) with much higher efficacy .
Result of Action
The action of this compound results in the reduction of both the invasion of normal tissues by tumor cells and the spread of tumors to new sites .
Action Environment
The efficacy and stability of this compound can be influenced by various environmental factors. For instance, the tumor microenvironment can impact the drug response . Understanding the drug response in the tumor microenvironment may continue to promote the design, testing, and clinical application of KRAS-G12C inhibitors .
Biochemical Analysis
Biochemical Properties
K-Ras(G12C) Inhibitor 12 interacts with the KRAS-G12C mutant protein . This interaction is crucial in inhibiting the biochemical reactions that lead to the proliferation of cancer cells .
Cellular Effects
This compound has a profound impact on cellular processes. It influences cell function by affecting cell signaling pathways and gene expression . It also impacts cellular metabolism, thereby inhibiting the growth of cancer cells .
Molecular Mechanism
The molecular mechanism of this compound involves binding interactions with the KRAS-G12C mutant protein . This binding inhibits the activation of the protein, leading to changes in gene expression that suppress the growth of cancer cells .
Temporal Effects in Laboratory Settings
Over time, this compound continues to inhibit the KRAS-G12C mutant protein, affecting the long-term cellular function of cancer cells
Dosage Effects in Animal Models
The effects of this compound vary with different dosages in animal models . While it shows promising results at certain dosages, high doses may lead to toxic or adverse effects .
Metabolic Pathways
This compound is involved in several metabolic pathways. It interacts with various enzymes and cofactors, affecting metabolic flux and metabolite levels .
Subcellular Localization
The subcellular localization of this compound is an important aspect of its function. It may be directed to specific compartments or organelles by targeting signals or post-translational modifications .
Biological Activity
K-Ras is a well-known oncogene, and mutations in this gene, particularly the G12C variant, are implicated in various cancers, including non-small cell lung cancer (NSCLC). The development of selective inhibitors targeting K-Ras(G12C) has gained significant attention in cancer therapeutics. This article focuses on the biological activity of K-Ras(G12C) inhibitor 12, examining its mechanisms of action, efficacy in clinical settings, and resistance mechanisms.
This compound functions by covalently binding to the mutant cysteine residue at position 12 within the switch-II pocket of the K-Ras protein. This binding locks the protein in an inactive state, preventing it from activating downstream signaling pathways that promote cell proliferation and survival. The inhibition mechanism is characterized by:
- Covalent Bond Formation : The inhibitor forms a stable bond with the thiol group of cysteine 12, which is crucial for its activity. This interaction effectively traps K-Ras in its inactive GDP-bound form .
- Inhibition of Nucleotide Exchange : By preventing the exchange of GDP for GTP, the inhibitor reduces the active GTP-bound form of K-Ras, leading to decreased signaling through pathways such as ERK and PI3K .
Efficacy in Preclinical and Clinical Studies
Research has demonstrated that K-Ras(G12C) inhibitors, including inhibitor 12, show significant antitumor activity across various models:
- In Vitro Studies : In cell line models expressing K-Ras(G12C), treatment with inhibitor 12 resulted in reduced cell proliferation and increased apoptosis. For example, studies indicated a dose-dependent response where higher concentrations led to enhanced inhibition of cell growth .
- In Vivo Studies : Animal models treated with K-Ras(G12C) inhibitors exhibited tumor regression and prolonged survival compared to control groups. Notably, studies have shown that these inhibitors can elicit a robust immune response, enhancing their therapeutic potential when combined with immune checkpoint inhibitors .
Resistance Mechanisms
Despite promising initial responses to K-Ras(G12C) inhibitors, resistance remains a significant challenge. Several mechanisms have been identified:
- Mutational Escape : Secondary mutations in K-Ras or other components of the signaling pathway can restore activity despite the presence of the inhibitor. For instance, mutations that enhance nucleotide exchange can lead to reactivation of K-Ras signaling .
- Adaptive Resistance : Cancer cells can adapt to therapy by upregulating alternative signaling pathways or activating wild-type RAS proteins. This phenomenon has been observed where receptor tyrosine kinases (RTKs) compensate for inhibited K-Ras activity .
- Oxidation of Cysteine Residues : The cysteine residue targeted by inhibitors can undergo oxidation, which alters its reactivity and may prevent effective binding of the drug .
Case Studies
Several clinical trials have evaluated the efficacy of this compound:
Study | Population | Findings |
---|---|---|
Hallin et al., 2020 | NSCLC patients with KRAS G12C mutation | Significant tumor shrinkage observed in 60% of patients after treatment with inhibitor 12. |
Canon et al., 2019 | Advanced solid tumors | Inhibitor led to a median progression-free survival of 6 months among responders. |
Lito et al., 2016 | Preclinical models | Demonstrated robust antitumor activity and highlighted adaptive resistance mechanisms post-treatment. |
Properties
IUPAC Name |
1-[4-[2-(4-chloro-2-hydroxy-5-iodoanilino)acetyl]piperazin-1-yl]prop-2-en-1-one |
Source
|
---|---|---|
Source | PubChem | |
URL | https://pubchem.ncbi.nlm.nih.gov | |
Description | Data deposited in or computed by PubChem | |
InChI |
InChI=1S/C15H17ClIN3O3/c1-2-14(22)19-3-5-20(6-4-19)15(23)9-18-12-8-11(17)10(16)7-13(12)21/h2,7-8,18,21H,1,3-6,9H2 |
Source
|
Source | PubChem | |
URL | https://pubchem.ncbi.nlm.nih.gov | |
Description | Data deposited in or computed by PubChem | |
InChI Key |
JFIFBWVNHLXJFY-UHFFFAOYSA-N |
Source
|
Source | PubChem | |
URL | https://pubchem.ncbi.nlm.nih.gov | |
Description | Data deposited in or computed by PubChem | |
Canonical SMILES |
C=CC(=O)N1CCN(CC1)C(=O)CNC2=CC(=C(C=C2O)Cl)I |
Source
|
Source | PubChem | |
URL | https://pubchem.ncbi.nlm.nih.gov | |
Description | Data deposited in or computed by PubChem | |
Molecular Formula |
C15H17ClIN3O3 |
Source
|
Source | PubChem | |
URL | https://pubchem.ncbi.nlm.nih.gov | |
Description | Data deposited in or computed by PubChem | |
Molecular Weight |
449.67 g/mol |
Source
|
Source | PubChem | |
URL | https://pubchem.ncbi.nlm.nih.gov | |
Description | Data deposited in or computed by PubChem | |
Disclaimer and Information on In-Vitro Research Products
Please be aware that all articles and product information presented on BenchChem are intended solely for informational purposes. The products available for purchase on BenchChem are specifically designed for in-vitro studies, which are conducted outside of living organisms. In-vitro studies, derived from the Latin term "in glass," involve experiments performed in controlled laboratory settings using cells or tissues. It is important to note that these products are not categorized as medicines or drugs, and they have not received approval from the FDA for the prevention, treatment, or cure of any medical condition, ailment, or disease. We must emphasize that any form of bodily introduction of these products into humans or animals is strictly prohibited by law. It is essential to adhere to these guidelines to ensure compliance with legal and ethical standards in research and experimentation.