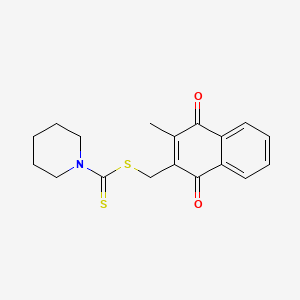
Pkm2-IN-1
- Click on QUICK INQUIRY to receive a quote from our team of experts.
- With the quality product at a COMPETITIVE price, you can focus more on your research.
Overview
Description
PKM2-IN-1 is a chemical compound known for its inhibitory effects on pyruvate kinase M2 (PKM2), an enzyme that plays a crucial role in the glycolytic pathway. PKM2 is involved in the conversion of phosphoenolpyruvate to pyruvate, a key step in cellular metabolism. This compound has been identified as a potential therapeutic agent due to its ability to modulate the activity of PKM2, making it a promising candidate for cancer treatment .
Mechanism of Action
Mode of Action
PKM2 inhibitors work by interacting with PKM2 and inhibiting its activity . The transition between PKM2 dimers and tetramers is allosterically regulated by endogenous and exogenous activators and inhibitors . PKM2 has PK enzyme activity only when it serves as a tetramer . PKM2 is activated by the glycolytic intermediate products named fructose 1,6-bisphosphate (FBP) . PKM2 inhibitors can prevent this activation, thereby inhibiting the enzyme’s activity and disrupting the glycolytic pathway .
Biochemical Pathways
PKM2 plays a significant role in various biological processes of tumor growth . It regulates the last step of the glycolytic pathway . The dimerization of PKM2 can promote the proliferation and growth of tumor cells . The aggregation of PKM2 is regulated by both endogenous and exogenous cofactors as well as post-translational modification (PTM) . PKM2 can also regulate each other with various proteins by phosphorylation, acetylation, and other modifications .
Result of Action
The inhibition of PKM2 can have several effects at the molecular and cellular levels. For instance, it can reduce reactive oxygen species (ROS) production and microbial killing by neutrophils . It can also slow down fibrosis progression . Moreover, the inhibition of PKM2 can lead to cell killing by apoptosis .
Action Environment
The action of PKM2 inhibitors can be influenced by various environmental factors. For example, the transition between PKM2 dimers and tetramers is allosterically regulated by endogenous and exogenous activators and inhibitors . Therefore, the presence of these activators and inhibitors in the cellular environment can influence the action of PKM2 inhibitors. Additionally, the pH and oxygen levels in the tumor microenvironment can also affect the activity of PKM2 and the efficacy of its inhibitors .
Biochemical Analysis
Biochemical Properties
The PKM2 inhibitor interacts with the PKM2 enzyme, which is involved in the final step of glycolysis . The inhibitor has an IC50 value of 2.95 μM, indicating its potent inhibitory effect on PKM2 . The interaction between the PKM2 inhibitor and the PKM2 enzyme is dose-dependent, with higher concentrations of the inhibitor leading to greater inhibition of the enzyme .
Cellular Effects
The PKM2 inhibitor impacts various cellular processes by influencing the function of the PKM2 enzyme. By inhibiting PKM2, the PKM2 inhibitor can disrupt glycolysis, leading to alterations in cellular metabolism . This can affect cell signaling pathways and gene expression, potentially influencing cell growth and proliferation .
Molecular Mechanism
The PKM2 inhibitor exerts its effects at the molecular level by binding to the PKM2 enzyme and inhibiting its activity . This interaction prevents the PKM2 enzyme from catalyzing the conversion of phosphoenolpyruvate to pyruvate, a key step in glycolysis . As a result, the PKM2 inhibitor can influence gene expression and metabolic processes within the cell .
Temporal Effects in Laboratory Settings
In laboratory settings, the effects of the PKM2 inhibitor can change over time. The stability and degradation of the PKM2 inhibitor can influence its long-term effects on cellular function
Dosage Effects in Animal Models
In animal models, the effects of the PKM2 inhibitor can vary with different dosages. Higher doses of the PKM2 inhibitor can lead to more pronounced inhibition of the PKM2 enzyme . High doses may also result in toxic or adverse effects, highlighting the importance of careful dosage control .
Metabolic Pathways
The PKM2 inhibitor is involved in the glycolytic pathway by interacting with the PKM2 enzyme . This interaction can affect metabolic flux and metabolite levels within the cell .
Subcellular Localization
The subcellular localization of the PKM2 inhibitor can influence its activity and function. The PKM2 inhibitor likely targets the cytoplasm, where the PKM2 enzyme is typically located
Preparation Methods
Synthetic Routes and Reaction Conditions: The synthesis of PKM2-IN-1 involves multiple steps, starting with the preparation of intermediate compounds. One common synthetic route includes the use of benzyl bromide and potassium tert-butoxide in dimethylformamide, followed by further reactions with tert-butyl 3-(bromomethyl)phenylcarbamate and hydrochloric acid in dioxane . The reaction conditions typically involve controlled temperatures and specific solvents to ensure the desired product yield.
Industrial Production Methods: Industrial production of this compound would likely involve scaling up the laboratory synthesis methods, optimizing reaction conditions for higher yields, and ensuring the purity of the final product. This may include the use of large-scale reactors, continuous flow systems, and advanced purification techniques such as chromatography.
Chemical Reactions Analysis
Types of Reactions: PKM2-IN-1 undergoes various chemical reactions, including oxidation, reduction, and substitution. These reactions are essential for modifying the compound’s structure and enhancing its inhibitory effects on PKM2.
Common Reagents and Conditions: Common reagents used in the reactions involving this compound include oxidizing agents like hydrogen peroxide, reducing agents such as sodium borohydride, and substitution reagents like alkyl halides. The reaction conditions often involve specific temperatures, pH levels, and solvents to achieve the desired chemical transformations .
Major Products Formed: The major products formed from the reactions involving this compound depend on the specific reagents and conditions used. For example, oxidation reactions may yield oxidized derivatives of this compound, while substitution reactions can produce various substituted analogs with enhanced biological activity.
Scientific Research Applications
Cancer Treatment
Mechanism of Action:
Pkm2-IN-1 activates PKM2, shifting the enzyme's equilibrium towards the tetrameric form, which enhances glycolytic flux and reduces lactate production. This shift can lead to decreased tumor growth and increased sensitivity to other chemotherapeutic agents.
Case Studies:
- Combination Therapy: In studies combining this compound with 2-deoxyglucose (2-DG), researchers observed enhanced inhibition of cancer cell proliferation across various tumor types, including lung and breast cancers. The combination therapy resulted in significantly lower glucose levels and increased lactate production, indicating heightened glycolytic activity .
- In Vivo Models: Animal models treated with this compound showed reduced tumor sizes compared to controls, demonstrating the compound's efficacy in vivo .
Metabolic Diseases
Role in Metabolic Reprogramming:
this compound has been investigated for its potential to ameliorate conditions related to metabolic diseases such as diabetes and obesity. Activation of PKM2 can enhance insulin sensitivity and promote better glucose homeostasis.
Research Findings:
- Diabetic Nephropathy: In experimental models of diabetic nephropathy, this compound treatment improved renal function and reduced inflammation associated with hyperglycemia .
- Inflammatory Responses: PKM2 activation has been linked to modulation of inflammatory pathways, suggesting that this compound could be beneficial in treating inflammatory diseases related to metabolic dysfunctions .
Data Tables
Application Area | Mechanism | Key Findings |
---|---|---|
Cancer Treatment | Activates PKM2 | Enhanced sensitivity to 2-DG; reduced tumor size in animal models |
Metabolic Diseases | Improves glucose metabolism | Improved renal function in diabetic models; reduced inflammation |
Immune Response Modulation | Regulates cytokine production | Inhibition of IL-1β secretion; potential therapeutic target for autoimmune diseases |
Comparison with Similar Compounds
PKM2-IN-1 is unique in its specific inhibition of PKM2, distinguishing it from other compounds that target different enzymes in the glycolytic pathway. Similar compounds include PKM2 activator 2, dimethylaminomicheliolide hydrochloride, and mitapivat, which also modulate the activity of PKM2 but through different mechanisms .
This compound stands out due to its high specificity and potency, with an IC50 value of 2.95 μM . This makes it a valuable tool for studying the role of PKM2 in various biological processes and for developing targeted therapies for diseases such as cancer.
Biological Activity
Pkm2-IN-1 is a small molecule inhibitor designed to target pyruvate kinase M2 (PKM2), a crucial enzyme in cellular metabolism and cancer biology. This article explores the biological activity of this compound, focusing on its mechanisms of action, effects on metabolic pathways, and implications for cancer therapy.
Overview of PKM2
PKM2 is a glycolytic enzyme that plays a significant role in regulating metabolic processes within cells. It exists in two forms: a tetrameric form that exhibits high enzymatic activity and a dimeric form that has lower activity. The balance between these forms is critical for cellular metabolism, particularly in cancer cells, where PKM2 supports the Warburg effect—favoring aerobic glycolysis over oxidative phosphorylation.
This compound inhibits PKM2 by stabilizing the dimeric form, thereby reducing its enzymatic activity and altering metabolic flux. This inhibition has several downstream effects:
- Reduction in Lactate Production : By inhibiting PKM2, this compound decreases lactate production, which is often elevated in cancer cells due to increased glycolysis.
- Altered Gene Expression : PKM2 influences the expression of various genes involved in cell proliferation and survival. Inhibition by this compound can lead to decreased expression of oncogenes such as MYC and CCND1, which are critical for tumor growth.
- Impact on Immune Response : PKM2 also plays a role in modulating immune responses. Inhibition may enhance anti-tumor immunity by altering the metabolic state of immune cells.
In Vitro Studies
In vitro studies have demonstrated that this compound effectively reduces PKM2 activity in various cancer cell lines. For example:
Cell Line | Effect of this compound | Reference |
---|---|---|
HeLa | Decreased lactate production | |
Hep3B | Reduced proliferation rates | |
A549 (Lung Cancer) | Inhibition of glycolytic flux |
These studies indicate that this compound can effectively disrupt the metabolic adaptations that cancer cells rely on for growth and survival.
Case Studies
A notable case study involved the use of this compound in a mouse model of breast cancer. The treatment led to:
- Tumor Growth Inhibition : Mice treated with this compound showed a significant reduction in tumor size compared to control groups.
- Increased Immune Cell Activation : There was an observed increase in CD8+ T cell activation, suggesting enhanced anti-tumor immunity.
Implications for Cancer Therapy
The biological activity of this compound highlights its potential as a therapeutic agent in cancer treatment. By targeting PKM2, this compound not only disrupts tumor metabolism but also enhances immune responses against tumors. This dual mechanism could provide a novel approach to overcoming resistance to traditional therapies.
Properties
IUPAC Name |
(3-methyl-1,4-dioxonaphthalen-2-yl)methyl piperidine-1-carbodithioate |
Source
|
---|---|---|
Source | PubChem | |
URL | https://pubchem.ncbi.nlm.nih.gov | |
Description | Data deposited in or computed by PubChem | |
InChI |
InChI=1S/C18H19NO2S2/c1-12-15(11-23-18(22)19-9-5-2-6-10-19)17(21)14-8-4-3-7-13(14)16(12)20/h3-4,7-8H,2,5-6,9-11H2,1H3 |
Source
|
Source | PubChem | |
URL | https://pubchem.ncbi.nlm.nih.gov | |
Description | Data deposited in or computed by PubChem | |
InChI Key |
STAFOGVMELKGRI-UHFFFAOYSA-N |
Source
|
Source | PubChem | |
URL | https://pubchem.ncbi.nlm.nih.gov | |
Description | Data deposited in or computed by PubChem | |
Canonical SMILES |
CC1=C(C(=O)C2=CC=CC=C2C1=O)CSC(=S)N3CCCCC3 |
Source
|
Source | PubChem | |
URL | https://pubchem.ncbi.nlm.nih.gov | |
Description | Data deposited in or computed by PubChem | |
Molecular Formula |
C18H19NO2S2 |
Source
|
Source | PubChem | |
URL | https://pubchem.ncbi.nlm.nih.gov | |
Description | Data deposited in or computed by PubChem | |
Molecular Weight |
345.5 g/mol |
Source
|
Source | PubChem | |
URL | https://pubchem.ncbi.nlm.nih.gov | |
Description | Data deposited in or computed by PubChem | |
Disclaimer and Information on In-Vitro Research Products
Please be aware that all articles and product information presented on BenchChem are intended solely for informational purposes. The products available for purchase on BenchChem are specifically designed for in-vitro studies, which are conducted outside of living organisms. In-vitro studies, derived from the Latin term "in glass," involve experiments performed in controlled laboratory settings using cells or tissues. It is important to note that these products are not categorized as medicines or drugs, and they have not received approval from the FDA for the prevention, treatment, or cure of any medical condition, ailment, or disease. We must emphasize that any form of bodily introduction of these products into humans or animals is strictly prohibited by law. It is essential to adhere to these guidelines to ensure compliance with legal and ethical standards in research and experimentation.