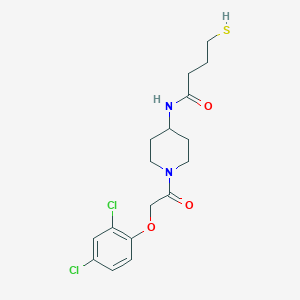
K-Ras(G12C) inhibitor 6
Overview
Description
K-Ras(G12C) inhibitor 6 is an irreversible inhibitor specifically targeting the oncogenic K-Ras(G12C) mutation. This mutation is a common driver in various cancers, including non-small cell lung cancer, colorectal cancer, and pancreatic cancer. The compound works by subverting the native nucleotide preference to favor guanosine diphosphate over guanosine triphosphate, thereby inhibiting the oncogenic activity of the K-Ras protein .
Scientific Research Applications
K-Ras(G12C) inhibitor 6 has significant applications in scientific research, particularly in the fields of chemistry, biology, and medicine. In chemistry, it is used as a model compound to study the mechanisms of irreversible inhibition. In biology, it serves as a tool to investigate the role of K-Ras mutations in cellular signaling pathways. In medicine, this compound is being explored as a potential therapeutic agent for treating cancers harboring the K-Ras(G12C) mutation. Clinical trials have shown promising results, with the compound demonstrating efficacy in reducing tumor growth and improving patient outcomes .
Mechanism of Action
Target of Action
The primary target of K-Ras(G12C) Inhibitor 6, also known as N-{1-[(2,4-Dichlorophenoxy)acetyl]piperidin-4-Yl}-4-Sulfanylbutanamide, is the oncogenic K-Ras(G12C) protein . This protein is a member of the RAS family and its mutations are genetic drivers of multiple cancer types, especially colorectal cancer, pancreatic ductal adenocarcinoma, and non-small cell lung cancer .
Mode of Action
This compound is an irreversible inhibitor of the oncogenic K-Ras(G12C) protein . It subverts the native nucleotide preference to favor GDP over GTP . The inhibitor allosterically controls GTP affinity and effector interactions by fitting inside a “pocket”, or binding site, of the mutant K-Ras . This action locks K-Ras in the inactive state, arresting cell proliferation .
Biochemical Pathways
Once activated, K-Ras activates multiple downstream pathways including the RAF–MEK–MAPK and PI3K–AKT–mTOR pathways which stimulate cell growth and survival . By inhibiting K-Ras(G12C), these pathways are suppressed, leading to a decrease in cell growth and survival .
Result of Action
The result of this compound’s action is the suppression of cell growth and survival in cells harboring the K-Ras(G12C) mutation . This can lead to a decrease in tumor size and potentially slow the progression of cancers driven by this mutation .
Action Environment
The efficacy and stability of this compound can be influenced by various environmental factors. For instance, the tumor microenvironment can impact drug response . Additionally, intrinsic or acquired resistance caused by cellular, molecular, and genetic mechanisms can also affect the efficacy of this compound . Understanding these factors is crucial for the design, testing, and clinical application of K-Ras(G12C) inhibitors .
Biochemical Analysis
Biochemical Properties
K-Ras(G12C) inhibitor 6 interacts with the KRAS protein, specifically the G12C mutation . This mutation introduces a cysteine residue that has strong nucleophilicity, allowing the inhibitor to bind covalently . The binding specificity of this compound is influenced by residues H95 and Y96 .
Cellular Effects
The compound exerts its effects on cells by inhibiting the KRAS protein, thereby affecting cell signaling pathways and gene expression . This can influence various cellular processes, including cellular metabolism .
Molecular Mechanism
This compound exerts its effects at the molecular level by binding to the GDP-bound state of KRAS (G12C), exploiting the strong nucleophilicity of the acquired cysteine . This binding interaction inhibits the activity of the KRAS protein, leading to changes in gene expression .
Temporal Effects in Laboratory Settings
The effects of this compound can change over time in laboratory settings. For example, oxidation of KRAS G12C can occur readily both in vitro and in the cellular environment, preventing the covalent binding of certain inhibitors .
Dosage Effects in Animal Models
The effects of this compound can vary with different dosages in animal models
Metabolic Pathways
Given its role in inhibiting the KRAS protein, it is likely that it interacts with enzymes and cofactors involved in cell signaling pathways .
Subcellular Localization
Given its target, the KRAS protein, it is likely that it localizes to the cell membrane where KRAS is typically found .
Preparation Methods
Synthetic Routes and Reaction Conditions: The synthesis of K-Ras(G12C) inhibitor 6 involves multiple steps, starting with the preparation of key intermediates. One common route includes the reaction of 2,4-dichlorophenoxyacetic acid with piperidine to form an intermediate, which is then further reacted with 4-sulfanylbutanamide under specific conditions to yield the final product .
Industrial Production Methods: Industrial production of this compound typically involves large-scale synthesis using optimized reaction conditions to ensure high yield and purity. The process includes rigorous purification steps such as recrystallization and chromatography to achieve the desired quality of the compound .
Chemical Reactions Analysis
Types of Reactions: K-Ras(G12C) inhibitor 6 primarily undergoes substitution reactions due to the presence of reactive functional groups such as the sulfanyl and amide groups. These reactions are crucial for its binding to the K-Ras protein.
Common Reagents and Conditions: Common reagents used in the synthesis and modification of this compound include dichlorophenoxyacetic acid, piperidine, and various solvents like dimethyl sulfoxide and acetonitrile. Reaction conditions often involve controlled temperatures and pH levels to ensure optimal reaction rates and product stability .
Major Products Formed: The major product formed from the reactions involving this compound is the final inhibitor compound itself, which is characterized by its high specificity and potency against the K-Ras(G12C) mutation .
Comparison with Similar Compounds
Properties
IUPAC Name |
N-[1-[2-(2,4-dichlorophenoxy)acetyl]piperidin-4-yl]-4-sulfanylbutanamide | |
---|---|---|
Source | PubChem | |
URL | https://pubchem.ncbi.nlm.nih.gov | |
Description | Data deposited in or computed by PubChem | |
InChI |
InChI=1S/C17H22Cl2N2O3S/c18-12-3-4-15(14(19)10-12)24-11-17(23)21-7-5-13(6-8-21)20-16(22)2-1-9-25/h3-4,10,13,25H,1-2,5-9,11H2,(H,20,22) | |
Source | PubChem | |
URL | https://pubchem.ncbi.nlm.nih.gov | |
Description | Data deposited in or computed by PubChem | |
InChI Key |
ZPXCEHMKUTXHRZ-UHFFFAOYSA-N | |
Source | PubChem | |
URL | https://pubchem.ncbi.nlm.nih.gov | |
Description | Data deposited in or computed by PubChem | |
Canonical SMILES |
C1CN(CCC1NC(=O)CCCS)C(=O)COC2=C(C=C(C=C2)Cl)Cl | |
Source | PubChem | |
URL | https://pubchem.ncbi.nlm.nih.gov | |
Description | Data deposited in or computed by PubChem | |
Molecular Formula |
C17H22Cl2N2O3S | |
Source | PubChem | |
URL | https://pubchem.ncbi.nlm.nih.gov | |
Description | Data deposited in or computed by PubChem | |
DSSTOX Substance ID |
DTXSID701143036 | |
Record name | Butanamide, N-[1-[2-(2,4-dichlorophenoxy)acetyl]-4-piperidinyl]-4-mercapto- | |
Source | EPA DSSTox | |
URL | https://comptox.epa.gov/dashboard/DTXSID701143036 | |
Description | DSSTox provides a high quality public chemistry resource for supporting improved predictive toxicology. | |
Molecular Weight |
405.3 g/mol | |
Source | PubChem | |
URL | https://pubchem.ncbi.nlm.nih.gov | |
Description | Data deposited in or computed by PubChem | |
CAS No. |
2060530-16-5 | |
Record name | Butanamide, N-[1-[2-(2,4-dichlorophenoxy)acetyl]-4-piperidinyl]-4-mercapto- | |
Source | CAS Common Chemistry | |
URL | https://commonchemistry.cas.org/detail?cas_rn=2060530-16-5 | |
Description | CAS Common Chemistry is an open community resource for accessing chemical information. Nearly 500,000 chemical substances from CAS REGISTRY cover areas of community interest, including common and frequently regulated chemicals, and those relevant to high school and undergraduate chemistry classes. This chemical information, curated by our expert scientists, is provided in alignment with our mission as a division of the American Chemical Society. | |
Explanation | The data from CAS Common Chemistry is provided under a CC-BY-NC 4.0 license, unless otherwise stated. | |
Record name | k-Ras(g12C) inhibitor 6 | |
Source | ChemIDplus | |
URL | https://pubchem.ncbi.nlm.nih.gov/substance/?source=chemidplus&sourceid=2060530165 | |
Description | ChemIDplus is a free, web search system that provides access to the structure and nomenclature authority files used for the identification of chemical substances cited in National Library of Medicine (NLM) databases, including the TOXNET system. | |
Record name | Butanamide, N-[1-[2-(2,4-dichlorophenoxy)acetyl]-4-piperidinyl]-4-mercapto- | |
Source | EPA DSSTox | |
URL | https://comptox.epa.gov/dashboard/DTXSID701143036 | |
Description | DSSTox provides a high quality public chemistry resource for supporting improved predictive toxicology. | |
Record name | K-RAS(G12C) INHIBITOR 6 | |
Source | FDA Global Substance Registration System (GSRS) | |
URL | https://gsrs.ncats.nih.gov/ginas/app/beta/substances/JPV55C3PN4 | |
Description | The FDA Global Substance Registration System (GSRS) enables the efficient and accurate exchange of information on what substances are in regulated products. Instead of relying on names, which vary across regulatory domains, countries, and regions, the GSRS knowledge base makes it possible for substances to be defined by standardized, scientific descriptions. | |
Explanation | Unless otherwise noted, the contents of the FDA website (www.fda.gov), both text and graphics, are not copyrighted. They are in the public domain and may be republished, reprinted and otherwise used freely by anyone without the need to obtain permission from FDA. Credit to the U.S. Food and Drug Administration as the source is appreciated but not required. | |
Disclaimer and Information on In-Vitro Research Products
Please be aware that all articles and product information presented on BenchChem are intended solely for informational purposes. The products available for purchase on BenchChem are specifically designed for in-vitro studies, which are conducted outside of living organisms. In-vitro studies, derived from the Latin term "in glass," involve experiments performed in controlled laboratory settings using cells or tissues. It is important to note that these products are not categorized as medicines or drugs, and they have not received approval from the FDA for the prevention, treatment, or cure of any medical condition, ailment, or disease. We must emphasize that any form of bodily introduction of these products into humans or animals is strictly prohibited by law. It is essential to adhere to these guidelines to ensure compliance with legal and ethical standards in research and experimentation.