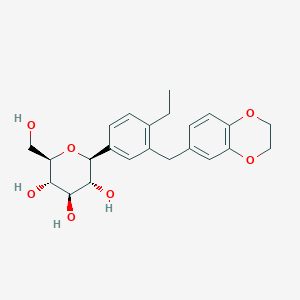
Licogliflozin
Overview
Description
Licogliflozin is a drug that acts as an inhibitor of sodium-glucose cotransporters 1 and 2. It was initially developed as a potential treatment for obesity but has also been investigated for other applications such as treating symptoms of polycystic ovary syndrome and nonalcoholic steatohepatitis .
Mechanism of Action
Target of Action
Licogliflozin is a dual inhibitor of sodium-glucose co-transporter 1 (SGLT1) and sodium-glucose co-transporter 2 (SGLT2) . These transporters play a crucial role in glucose reabsorption in the kidneys (SGLT2) and the intestines (SGLT1) .
Mode of Action
This compound works by inhibiting SGLT1 and SGLT2, thereby reducing the reabsorption of glucose in the kidneys and intestines . This leads to an increase in glucose excretion through urine and a decrease in blood glucose levels .
Biochemical Pathways
The inhibition of SGLT1 and SGLT2 by this compound affects several biochemical pathways. It impacts lipid and glucose metabolism, iron handling, and cardiomyocyte function . The most significant changes were detected in protein species potentially related to the clinical and metabolic changes that were actually measured in the same patients .
Pharmacokinetics
The pharmacokinetics of this compound involve its absorption, distribution, metabolism, and excretion (ADME). It’s known that the elimination of this compound is predominantly via metabolism, with the majority of the dose excreted as metabolites . The principal biotransformation pathways involved direct glucuronidation and oxidation .
Result of Action
This compound has been shown to significantly reduce body weight and improve metabolic parameters . It reduces postprandial glucose excursion, insulin levels, and increases glucagon . In patients with type 2 diabetes mellitus (T2DM), a single dose of this compound remarkably reduced glucose excursion and suppressed insulin .
Action Environment
Environmental factors such as diet and lifestyle can influence the action of this compound. For instance, in overweight or obese women with polycystic ovary syndrome (PCOS), this compound improved glucolipid metabolism and reproductive hormone status . .
Biochemical Analysis
Biochemical Properties
Licogliflozin is a dual SGLT1/2 inhibitor acting on the intestine and kidney by reducing glycemic and calorie content . It interacts with the SGLT1 and SGLT2 proteins, which are responsible for glucose reabsorption in the kidneys . The inhibition of these proteins leads to a decrease in blood glucose levels .
Cellular Effects
This compound has been shown to have significant effects on various types of cells and cellular processes. For instance, it has been found to improve left atrial dysfunction in a metabolic syndrome-related rat model of HFpEF . It also has a significant impact on cell signaling pathways, gene expression, and cellular metabolism .
Molecular Mechanism
The molecular mechanism of this compound involves its action as an SGLT2 inhibitor . It binds to the SGLT2 protein, inhibiting its function and leading to a decrease in glucose reabsorption in the kidneys . This results in a decrease in blood glucose levels .
Temporal Effects in Laboratory Settings
In laboratory settings, this compound has shown significant effects over time. For instance, after 12 weeks of treatment with this compound 150 mg, there was a significant 32% placebo-adjusted reduction in serum ALT . This indicates the long-term effects of this compound on cellular function in in vitro or in vivo studies.
Metabolic Pathways
This compound is involved in the metabolic pathway of glucose reabsorption in the kidneys . It interacts with the SGLT1 and SGLT2 proteins, which are key enzymes in this pathway . The inhibition of these proteins leads to a decrease in glucose reabsorption, affecting metabolic flux and metabolite levels .
Transport and Distribution
Given its role as an SGLT2 inhibitor, it can be inferred that it is likely transported to the kidneys where it exerts its effects .
Subcellular Localization
Given its role as an SGLT2 inhibitor, it can be inferred that it likely localizes to the cell membrane where the SGLT2 proteins are located .
Preparation Methods
Synthetic Routes and Reaction Conditions
Licogliflozin is synthesized through a multi-step process involving the formation of a benzodioxin ring and subsequent glycosylation. The key steps include:
Formation of the benzodioxin ring: This involves the reaction of a suitable phenol derivative with an appropriate diol under acidic conditions.
Glycosylation: The benzodioxin intermediate is then glycosylated using a suitable glycosyl donor in the presence of a Lewis acid catalyst.
Industrial Production Methods
The industrial production of this compound involves scaling up the synthetic route with optimization of reaction conditions to ensure high yield and purity. This includes:
Optimization of reaction conditions: Ensuring the reactions are carried out under controlled temperature and pressure to maximize yield.
Purification: Using techniques such as crystallization and chromatography to purify the final product.
Chemical Reactions Analysis
Types of Reactions
Licogliflozin undergoes several types of chemical reactions, including:
Oxidation: It can be oxidized to form various metabolites.
Reduction: Reduction reactions can modify the functional groups present in the molecule.
Substitution: Various substitution reactions can occur, particularly on the aromatic ring.
Common Reagents and Conditions
Oxidation: Common oxidizing agents include potassium permanganate and hydrogen peroxide.
Reduction: Reducing agents such as sodium borohydride and lithium aluminum hydride are used.
Substitution: Conditions vary depending on the substituent being introduced, but common reagents include halogens and nucleophiles.
Major Products
The major products formed from these reactions include various oxidized and reduced metabolites, as well as substituted derivatives of this compound .
Scientific Research Applications
Chemistry: Used as a model compound to study the inhibition of sodium-glucose cotransporters.
Biology: Investigated for its effects on glucose metabolism and weight reduction.
Medicine: Explored as a treatment for obesity, polycystic ovary syndrome, and nonalcoholic steatohepatitis.
Industry: Potential applications in the development of new therapeutic agents targeting metabolic disorders.
Comparison with Similar Compounds
Licogliflozin is compared with other sodium-glucose cotransporter inhibitors such as canagliflozin, empagliflozin, and sotagliflozin. While all these compounds inhibit sodium-glucose cotransporter 2, this compound also inhibits sodium-glucose cotransporter 1, making it unique in its dual inhibition mechanism. This dual inhibition results in more efficient glucose excretion and weight reduction compared to compounds that only inhibit sodium-glucose cotransporter 2 .
List of Similar Compounds
- Canagliflozin
- Empagliflozin
- Sotagliflozin
This compound’s dual inhibition of sodium-glucose cotransporters 1 and 2 sets it apart from these similar compounds, offering potential advantages in therapeutic applications .
Properties
IUPAC Name |
(2S,3R,4R,5S,6R)-2-[3-(2,3-dihydro-1,4-benzodioxin-6-ylmethyl)-4-ethylphenyl]-6-(hydroxymethyl)oxane-3,4,5-triol | |
---|---|---|
Source | PubChem | |
URL | https://pubchem.ncbi.nlm.nih.gov | |
Description | Data deposited in or computed by PubChem | |
InChI |
InChI=1S/C23H28O7/c1-2-14-4-5-15(23-22(27)21(26)20(25)19(12-24)30-23)11-16(14)9-13-3-6-17-18(10-13)29-8-7-28-17/h3-6,10-11,19-27H,2,7-9,12H2,1H3/t19-,20-,21+,22-,23+/m1/s1 | |
Source | PubChem | |
URL | https://pubchem.ncbi.nlm.nih.gov | |
Description | Data deposited in or computed by PubChem | |
InChI Key |
XFJAMQQAAMJFGB-ZQGJOIPISA-N | |
Source | PubChem | |
URL | https://pubchem.ncbi.nlm.nih.gov | |
Description | Data deposited in or computed by PubChem | |
Canonical SMILES |
CCC1=C(C=C(C=C1)C2C(C(C(C(O2)CO)O)O)O)CC3=CC4=C(C=C3)OCCO4 | |
Source | PubChem | |
URL | https://pubchem.ncbi.nlm.nih.gov | |
Description | Data deposited in or computed by PubChem | |
Isomeric SMILES |
CCC1=C(C=C(C=C1)[C@H]2[C@@H]([C@H]([C@@H]([C@H](O2)CO)O)O)O)CC3=CC4=C(C=C3)OCCO4 | |
Source | PubChem | |
URL | https://pubchem.ncbi.nlm.nih.gov | |
Description | Data deposited in or computed by PubChem | |
Molecular Formula |
C23H28O7 | |
Source | PubChem | |
URL | https://pubchem.ncbi.nlm.nih.gov | |
Description | Data deposited in or computed by PubChem | |
Molecular Weight |
416.5 g/mol | |
Source | PubChem | |
URL | https://pubchem.ncbi.nlm.nih.gov | |
Description | Data deposited in or computed by PubChem | |
CAS No. |
1291094-73-9 | |
Record name | Licogliflozin [INN] | |
Source | ChemIDplus | |
URL | https://pubchem.ncbi.nlm.nih.gov/substance/?source=chemidplus&sourceid=1291094739 | |
Description | ChemIDplus is a free, web search system that provides access to the structure and nomenclature authority files used for the identification of chemical substances cited in National Library of Medicine (NLM) databases, including the TOXNET system. | |
Record name | Licogliflozin | |
Source | DrugBank | |
URL | https://www.drugbank.ca/drugs/DB15048 | |
Description | The DrugBank database is a unique bioinformatics and cheminformatics resource that combines detailed drug (i.e. chemical, pharmacological and pharmaceutical) data with comprehensive drug target (i.e. sequence, structure, and pathway) information. | |
Explanation | Creative Common's Attribution-NonCommercial 4.0 International License (http://creativecommons.org/licenses/by-nc/4.0/legalcode) | |
Record name | LICOGLIFLOZIN | |
Source | FDA Global Substance Registration System (GSRS) | |
URL | https://gsrs.ncats.nih.gov/ginas/app/beta/substances/57J06X6EI0 | |
Description | The FDA Global Substance Registration System (GSRS) enables the efficient and accurate exchange of information on what substances are in regulated products. Instead of relying on names, which vary across regulatory domains, countries, and regions, the GSRS knowledge base makes it possible for substances to be defined by standardized, scientific descriptions. | |
Explanation | Unless otherwise noted, the contents of the FDA website (www.fda.gov), both text and graphics, are not copyrighted. They are in the public domain and may be republished, reprinted and otherwise used freely by anyone without the need to obtain permission from FDA. Credit to the U.S. Food and Drug Administration as the source is appreciated but not required. | |
Disclaimer and Information on In-Vitro Research Products
Please be aware that all articles and product information presented on BenchChem are intended solely for informational purposes. The products available for purchase on BenchChem are specifically designed for in-vitro studies, which are conducted outside of living organisms. In-vitro studies, derived from the Latin term "in glass," involve experiments performed in controlled laboratory settings using cells or tissues. It is important to note that these products are not categorized as medicines or drugs, and they have not received approval from the FDA for the prevention, treatment, or cure of any medical condition, ailment, or disease. We must emphasize that any form of bodily introduction of these products into humans or animals is strictly prohibited by law. It is essential to adhere to these guidelines to ensure compliance with legal and ethical standards in research and experimentation.