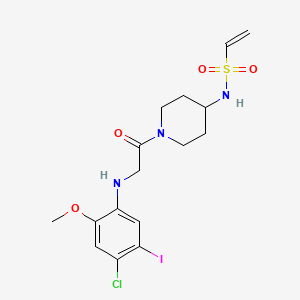
K-Ras(G12C) inhibitor 9
- Click on QUICK INQUIRY to receive a quote from our team of experts.
- With the quality product at a COMPETITIVE price, you can focus more on your research.
Overview
Description
K-Ras(G12C) inhibitor 9 is an allosteric inhibitor of oncogenic K-Ras(G12C) . It belongs to a series of small molecules that irreversibly bind to a common oncogenic mutant K-Ras(G12C) and block K-Ras(G12C) interactions . Some of these molecules decrease viability and increase apoptosis of G12C-containing cancer cell lines .
Chemical Reactions Analysis
K-Ras(G12C) inhibitor 9 is known to bind irreversibly to the mutant cysteine residue in oncogenic KRAS G12C . This mechanism of action surmounts the pharmacological problem of attempting to competitively inhibit GTP/GDP binding which occurs with picomolar affinities at RAS proteins .Scientific Research Applications
Oncology: Treatment of Non-Small Cell Lung Cancer (NSCLC)
The K-Ras(G12C) inhibitor 9 has shown promising improvements in clinical trials for the treatment of advanced non-small cell lung cancer (NSCLC) carrying KRAS-G12C mutations . Unfortunately, the majority of patients do not respond to KRAS-G12C inhibitor therapy due to intrinsic or acquired resistance caused by cellular, molecular, and genetic mechanisms .
Oncology: Treatment of Colorectal Cancer (CRC)
The KRAS gene, which the K-Ras(G12C) inhibitor 9 targets, is a genetic driver of multiple cancer types, especially colorectal cancer (CRC) . The inhibitor has potential applications in the treatment of CRCs with KRAS mutations .
Oncology: Treatment of Pancreatic Ductal Adenocarcinoma (PDAC)
The KRAS gene is also implicated in about 73% of pancreatic ductal adenocarcinoma (PDAC) cases . Therefore, the K-Ras(G12C) inhibitor 9 could be a potential therapeutic avenue for PDAC treatment .
Drug Resistance Research
Understanding the resistance to K-Ras(G12C) inhibitor 9 is crucial for the development of enhanced therapeutics and innovative drug combinations . Research in this area focuses on both direct resistance mechanisms, such as KRAS mutations that reduce drug efficacy, and indirect resistance mechanisms that rescue mutant KRAS-dependent cells .
Immunotherapy
The K-Ras(G12C) inhibitor 9 could potentially be used in immunostimulatory strategies targeting the KRAS-G12C mutation . This could revolutionize the treatment of certain cancer patients .
Development of Bispecific Antibodies
Research has shown that covalent K-Ras(G12C) inhibitors give rise to chemically modified cancer neoepitopes . This has led to the development of bispecific antibodies that induce T cell-mediated killing of KRAS G12C mutant cells .
Mechanism of Action
Target of Action
The primary target of K-Ras(G12C) inhibitor 9 is the K-Ras protein , specifically the G12C mutation . K-Ras is a small GTPase and is among the most commonly mutated oncogenes in cancer . The G12C mutation is particularly prevalent in non-small cell lung cancers (NSCLC), colorectal cancers (CRC), and pancreatic ductal adenocarcinoma (PDAC) .
Mode of Action
K-Ras(G12C) inhibitors, including inhibitor 9, preferentially bind to the GDP-bound conformation of the K-Ras protein . This binding blocks the exchange with GTP, thus preventing the activation of the signaling cascade . The inhibitors are covalent, meaning they form a strong bond with the mutant protein, locking it in an inactive state .
Biochemical Pathways
The action of K-Ras(G12C) inhibitors affects several biochemical pathways. The primary pathway is the RAS/MAPK pathway , which is involved in cell proliferation and survival . By inhibiting the G12C mutation, these inhibitors disrupt this pathway, leading to a reduction in oncogenic signaling . Resistance to these inhibitors can arise through various mechanisms, including reactivation of the same signaling or via alternative pathways .
Pharmacokinetics
The pharmacokinetics of K-Ras(G12C) inhibitors like inhibitor 9 involve their absorption, distribution, metabolism, and excretion (ADME). These properties impact the bioavailability of the drug. For example, GEC255, a novel KRAS G12C inhibitor, showed a linear increase in AUC exposure and Cmax with increasing doses . The Tmax was 2-4 hours in all dosing groups, and Ctrough within the 24-hour period was higher than pERK IC90 even in low dose group .
Result of Action
The result of the action of K-Ras(G12C) inhibitors is a decrease in the proliferation of cancer cells. This is achieved by locking the K-Ras protein in an inactive state, thus preventing it from promoting cell proliferation . Resistance to these inhibitors can develop through various mechanisms, including mutations that reduce drug efficacy or increase the expression of mutant k-ras .
Action Environment
The action, efficacy, and stability of K-Ras(G12C) inhibitors can be influenced by various environmental factors. These include the tumor microenvironment, which can impact drug response . For example, immune checkpoints, which are modulators of immune activation, can destroy anti-tumor immunity and thus influence the effectiveness of these inhibitors . Understanding these environmental influences is crucial for the design, testing, and clinical application of K-Ras(G12C) inhibitors .
Safety and Hazards
properties
IUPAC Name |
N-[1-[2-(4-chloro-5-iodo-2-methoxyanilino)acetyl]piperidin-4-yl]ethenesulfonamide |
Source
|
---|---|---|
Source | PubChem | |
URL | https://pubchem.ncbi.nlm.nih.gov | |
Description | Data deposited in or computed by PubChem | |
InChI |
InChI=1S/C16H21ClIN3O4S/c1-3-26(23,24)20-11-4-6-21(7-5-11)16(22)10-19-14-9-13(18)12(17)8-15(14)25-2/h3,8-9,11,19-20H,1,4-7,10H2,2H3 |
Source
|
Source | PubChem | |
URL | https://pubchem.ncbi.nlm.nih.gov | |
Description | Data deposited in or computed by PubChem | |
InChI Key |
ZGUSBCDCZNBNQT-UHFFFAOYSA-N |
Source
|
Source | PubChem | |
URL | https://pubchem.ncbi.nlm.nih.gov | |
Description | Data deposited in or computed by PubChem | |
Canonical SMILES |
COC1=CC(=C(C=C1NCC(=O)N2CCC(CC2)NS(=O)(=O)C=C)I)Cl |
Source
|
Source | PubChem | |
URL | https://pubchem.ncbi.nlm.nih.gov | |
Description | Data deposited in or computed by PubChem | |
Molecular Formula |
C16H21ClIN3O4S |
Source
|
Source | PubChem | |
URL | https://pubchem.ncbi.nlm.nih.gov | |
Description | Data deposited in or computed by PubChem | |
Molecular Weight |
513.8 g/mol |
Source
|
Source | PubChem | |
URL | https://pubchem.ncbi.nlm.nih.gov | |
Description | Data deposited in or computed by PubChem | |
Product Name |
K-Ras(G12C) inhibitor 9 |
Disclaimer and Information on In-Vitro Research Products
Please be aware that all articles and product information presented on BenchChem are intended solely for informational purposes. The products available for purchase on BenchChem are specifically designed for in-vitro studies, which are conducted outside of living organisms. In-vitro studies, derived from the Latin term "in glass," involve experiments performed in controlled laboratory settings using cells or tissues. It is important to note that these products are not categorized as medicines or drugs, and they have not received approval from the FDA for the prevention, treatment, or cure of any medical condition, ailment, or disease. We must emphasize that any form of bodily introduction of these products into humans or animals is strictly prohibited by law. It is essential to adhere to these guidelines to ensure compliance with legal and ethical standards in research and experimentation.