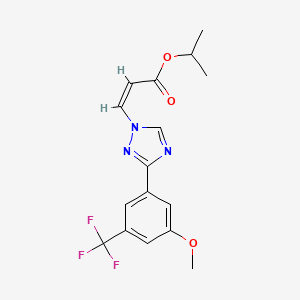
KPT-185
Overview
Description
This compound has shown significant potential in preclinical studies for its anticancer properties, particularly in the treatment of acute myeloid leukemia and other malignancies . KPT-185 works by blocking the nuclear export of tumor suppressor proteins, thereby restoring their function and inducing apoptosis in cancer cells .
Mechanism of Action
Target of Action
The primary target of KPT-185 is Chromosome Region Maintenance 1 (CRM1) , also known as Exportin 1 (XPO1) . CRM1 is a nuclear export receptor involved in the active transport of tumor suppressor proteins .
Mode of Action
This compound functions as a selective inhibitor of CRM1 . It binds to CRM1 within the Cys528 site, preventing CRM1 from interacting with tumor-suppressor proteins . This leads to the nuclear retention of these proteins, thereby restoring their tumor suppressor function .
Biochemical Pathways
The inhibition of CRM1 by this compound affects several biochemical pathways. One significant pathway involves the degradation of Notch1, a protein involved in cell differentiation, through the nuclear retention of Fbw7 . This leads to a decrease in tumor-promoting markers such as C-Myc, Cyclin-D1, Hes1, and VEGF .
Pharmacokinetics
This compound displays potent antiproliferative properties at submicromolar concentrations, with IC50 values ranging from 100-500 nM . It is orally bioavailable, making it a convenient option for administration .
Result of Action
The action of this compound results in significant molecular and cellular effects. It inhibits cell growth, induces apoptosis, and causes cell-cycle arrest in the G1 phase . Furthermore, it promotes myeloid differentiation in acute myeloid leukemia (AML) cell lines and patient blasts .
Biochemical Analysis
Biochemical Properties
KPT-185 interacts with CRM1, a key protein involved in the nuclear export of numerous molecules . By inhibiting CRM1, this compound disrupts the normal function of this protein, leading to a variety of biochemical effects . The compound has been shown to reduce the proliferation of patient-derived acute myeloid leukemia cells .
Cellular Effects
This compound has significant effects on various types of cells. It induces cell cycle arrest in the G1 phase and apoptosis in MOLT-4 T cell acute lymphoblastic leukemia cells and in a panel of six non-small cell lung cancer (NSCLC) cell lines . It also reduces the levels of CRM1 protein in the nucleus of these cells .
Molecular Mechanism
The molecular mechanism of this compound involves its binding to CRM1, inhibiting its function and leading to the accumulation of CRM1 cargo proteins in the nucleus . This results in changes in gene expression and cellular function .
Temporal Effects in Laboratory Settings
Over time, this compound continues to exert its effects on cells, leading to long-term changes in cellular function
Preparation Methods
Synthetic Routes and Reaction Conditions
KPT-185 is synthesized through a series of chemical reactions involving the formation of a triazole ring and the introduction of a trifluoromethyl group. The key steps in the synthesis include:
Formation of the triazole ring: This involves the reaction of an appropriate hydrazine derivative with a suitable nitrile compound under acidic conditions.
Introduction of the trifluoromethyl group: This step involves the reaction of the triazole intermediate with a trifluoromethylating agent, such as trifluoromethyl iodide, in the presence of a base.
Industrial Production Methods
The industrial production of this compound follows similar synthetic routes but is optimized for large-scale production. This includes the use of continuous flow reactors and automated systems to ensure consistent quality and yield. The compound is typically purified using chromatographic techniques and crystallization .
Chemical Reactions Analysis
Types of Reactions
KPT-185 undergoes several types of chemical reactions, including:
Oxidation: this compound can be oxidized to form various oxidation products, depending on the conditions used.
Reduction: The compound can be reduced to form different reduction products.
Common Reagents and Conditions
Oxidation: Common oxidizing agents include hydrogen peroxide and potassium permanganate.
Reduction: Reducing agents such as sodium borohydride and lithium aluminum hydride are commonly used.
Substitution: Nucleophiles such as amines, thiols, and alcohols can be used in substitution reactions.
Major Products
The major products formed from these reactions depend on the specific reagents and conditions used. For example, oxidation of this compound can lead to the formation of various oxidized derivatives, while reduction can yield different reduced forms of the compound .
Scientific Research Applications
KPT-185 has a wide range of scientific research applications, including:
Chemistry: this compound is used as a tool compound to study the role of nuclear export in various cellular processes.
Biology: In biological research, this compound is used to investigate the effects of nuclear export inhibition on cell cycle regulation, apoptosis, and differentiation.
Medicine: this compound has shown promising results in preclinical studies for the treatment of various cancers, including acute myeloid leukemia, non-small cell lung cancer, and pancreatic cancer.
Industry: In the pharmaceutical industry, this compound is used in the development of new anticancer drugs.
Comparison with Similar Compounds
KPT-185 belongs to a class of compounds known as selective inhibitors of nuclear export (SINE). Other similar compounds include:
KPT-330 (Selinexor): An oral SINE compound that has been approved for the treatment of multiple myeloma and diffuse large B cell lymphoma.
KPT-335 (Verdinexor): Another SINE compound that has shown efficacy in preclinical models of neuroblastoma and other cancers.
KPT-350:
Uniqueness of this compound
This compound is unique in its high potency and selectivity for CRM1 inhibition. It has demonstrated significant anticancer activity in various preclinical models, making it a valuable tool for studying the role of nuclear export in cancer and other diseases . Additionally, this compound’s irreversible binding to CRM1 sets it apart from other SINE compounds, which may have reversible binding properties .
Biological Activity
KPT-185 is a selective inhibitor of nuclear export (SINE) that targets exportin 1 (XPO1), a protein involved in the nuclear transport of various oncogenic factors. This compound has garnered attention for its potential therapeutic applications in various cancers, particularly those resistant to conventional treatments. The biological activity of this compound has been investigated through multiple studies, revealing its mechanisms of action, efficacy, and potential clinical applications.
This compound functions primarily by inhibiting XPO1, which is crucial for the nucleocytoplasmic transport of proteins and RNA. By blocking this pathway, this compound disrupts the export of several key regulatory proteins involved in cell proliferation and survival, leading to anti-proliferative effects in cancer cells.
Key Findings on Mechanism:
- Inhibition of Ribosomal Biogenesis : this compound has been shown to suppress ribosomal biogenesis in mantle cell lymphoma (MCL) cells, contributing to its anti-tumor effects. This inhibition is associated with decreased expression of oncogenic mediators such as cyclin D1 and c-Myc, and downregulation of chaperone proteins like HSP70 .
- Induction of Apoptosis : The compound induces apoptosis in cancer cells through p53-independent pathways, making it relevant for tumors with dysfunctional p53 signaling .
In Vitro Studies
This compound has demonstrated significant cytotoxicity across various cancer cell lines. The compound's effectiveness is often assessed using cell viability assays and apoptosis detection methods.
Table 1: Cytotoxicity Data of this compound
Cell Line | IC50 (µM) | Mechanism of Action |
---|---|---|
MCL Cells | 86.03 | Inhibition of XPO1, apoptosis induction |
A549 (RSV-infected) | 1.3 | Disruption of viral replication |
Ovarian Cancer Cells | Varies | Synergistic effect with cisplatin |
In Vivo Studies
Preclinical models have shown that this compound can significantly inhibit tumor growth when administered in vivo. For instance, studies involving patient-derived xenograft (PDX) models have indicated that this compound enhances the efficacy of standard chemotherapy agents like cisplatin .
Case Study 1: Mantle Cell Lymphoma
In a study focusing on MCL, this compound was administered to evaluate its impact on tumor growth and survival rates. Results indicated a marked reduction in tumor size and increased apoptosis in treated groups compared to controls. The study highlighted the compound's ability to target multiple oncogenic pathways simultaneously .
Case Study 2: Ovarian Cancer
A clinical trial involving patients with late-stage ovarian cancer treated with this compound showed promising results. Patients exhibited improved outcomes when this compound was combined with traditional chemotherapy, suggesting a synergistic effect that warrants further investigation .
Properties
IUPAC Name |
propan-2-yl (Z)-3-[3-[3-methoxy-5-(trifluoromethyl)phenyl]-1,2,4-triazol-1-yl]prop-2-enoate | |
---|---|---|
Source | PubChem | |
URL | https://pubchem.ncbi.nlm.nih.gov | |
Description | Data deposited in or computed by PubChem | |
InChI |
InChI=1S/C16H16F3N3O3/c1-10(2)25-14(23)4-5-22-9-20-15(21-22)11-6-12(16(17,18)19)8-13(7-11)24-3/h4-10H,1-3H3/b5-4- | |
Source | PubChem | |
URL | https://pubchem.ncbi.nlm.nih.gov | |
Description | Data deposited in or computed by PubChem | |
InChI Key |
NLNGWFLRRRYNIL-PLNGDYQASA-N | |
Source | PubChem | |
URL | https://pubchem.ncbi.nlm.nih.gov | |
Description | Data deposited in or computed by PubChem | |
Canonical SMILES |
CC(C)OC(=O)C=CN1C=NC(=N1)C2=CC(=CC(=C2)OC)C(F)(F)F | |
Source | PubChem | |
URL | https://pubchem.ncbi.nlm.nih.gov | |
Description | Data deposited in or computed by PubChem | |
Isomeric SMILES |
CC(C)OC(=O)/C=C\N1C=NC(=N1)C2=CC(=CC(=C2)OC)C(F)(F)F | |
Source | PubChem | |
URL | https://pubchem.ncbi.nlm.nih.gov | |
Description | Data deposited in or computed by PubChem | |
Molecular Formula |
C16H16F3N3O3 | |
Source | PubChem | |
URL | https://pubchem.ncbi.nlm.nih.gov | |
Description | Data deposited in or computed by PubChem | |
Molecular Weight |
355.31 g/mol | |
Source | PubChem | |
URL | https://pubchem.ncbi.nlm.nih.gov | |
Description | Data deposited in or computed by PubChem | |
Retrosynthesis Analysis
AI-Powered Synthesis Planning: Our tool employs the Template_relevance Pistachio, Template_relevance Bkms_metabolic, Template_relevance Pistachio_ringbreaker, Template_relevance Reaxys, Template_relevance Reaxys_biocatalysis model, leveraging a vast database of chemical reactions to predict feasible synthetic routes.
One-Step Synthesis Focus: Specifically designed for one-step synthesis, it provides concise and direct routes for your target compounds, streamlining the synthesis process.
Accurate Predictions: Utilizing the extensive PISTACHIO, BKMS_METABOLIC, PISTACHIO_RINGBREAKER, REAXYS, REAXYS_BIOCATALYSIS database, our tool offers high-accuracy predictions, reflecting the latest in chemical research and data.
Strategy Settings
Precursor scoring | Relevance Heuristic |
---|---|
Min. plausibility | 0.01 |
Model | Template_relevance |
Template Set | Pistachio/Bkms_metabolic/Pistachio_ringbreaker/Reaxys/Reaxys_biocatalysis |
Top-N result to add to graph | 6 |
Feasible Synthetic Routes
Q1: What is the primary target of KPT-185?
A1: this compound specifically targets Chromosome Region Maintenance 1 (CRM1), also known as Exportin 1 (XPO1), a key protein responsible for transporting various molecules, including tumor suppressor proteins (TSPs), from the nucleus to the cytoplasm. [, , , , , , , , , ]
Q2: How does this compound interact with CRM1?
A2: this compound irreversibly binds to CRM1, blocking its ability to recognize and bind to cargo proteins carrying a specific nuclear export signal (NES). This effectively traps these cargo proteins, many of which are TSPs, within the nucleus. [, , , , , , , , , ]
Q3: What are the downstream effects of this compound-mediated CRM1 inhibition?
A3: By blocking CRM1, this compound leads to the nuclear accumulation of various TSPs, including p53, FOXO3A, p21, IκB, and others. This accumulation allows these TSPs to exert their regulatory effects within the nucleus, ultimately leading to:
- Inhibition of cell proliferation: this compound disrupts cell cycle progression, primarily by inducing cell cycle arrest at the G1/S checkpoint. [, , , ]
- Induction of apoptosis: The nuclear accumulation of TSPs like p53 activates downstream apoptotic pathways, leading to programmed cell death. [, , , , , , , , ]
- Inhibition of oncogenic signaling pathways: this compound downregulates various oncogenic pathways, including NFκB, PI3K/Akt, and mTOR signaling. [, , , , ]
- Modulation of cellular metabolism: Research suggests that this compound, particularly in combination with mTOR inhibitors, can influence cellular metabolism by impacting pathways like ribosomal biogenesis, glycolysis, and the TCA cycle. [, ]
Q4: Does this compound affect normal cells?
A4: While this compound predominantly targets cancer cells, some studies show that it can also affect normal cells, albeit at much higher concentrations. The differential sensitivity is attributed to the higher dependence of cancer cells on CRM1-mediated nuclear export for survival and proliferation. [, , , ]
Q5: Does this compound influence Epithelial-to-Mesenchymal Transition (EMT)?
A5: Research suggests that this compound can reverse EMT in certain models. It accomplishes this by inducing nuclear retention of F-box protein FBXL5, which promotes the degradation of Snail, a key transcription factor involved in EMT. []
Q6: What is the molecular formula and weight of this compound?
A6: The molecular formula of this compound is C15H15F3N3O3, and its molecular weight is 343.3 g/mol.
Q7: How do structural modifications affect the activity of this compound analogs?
A7: While specific structure-activity relationships for this compound analogs were not extensively discussed in the analyzed papers, research mentions that mutations in the CRM1 binding site for SINE compounds, particularly at Cys-528, can significantly impact their efficacy. This highlights the importance of this specific interaction for this compound's activity. [, ]
Q8: What types of cancer cell lines have shown sensitivity to this compound in vitro?
A8: In vitro studies demonstrate the efficacy of this compound against a wide range of cancer cell lines, including:
- Hematological malignancies: Mantle Cell Lymphoma (MCL), Acute Myeloid Leukemia (AML), Multiple Myeloma (MM), Chronic Lymphocytic Leukemia (CLL) [, , , , , , , , , ]
- Solid tumors: Non-Small Cell Lung Cancer (NSCLC), Colon Cancer, Pancreatic Cancer, Ovarian Cancer, Melanoma, Breast Cancer, Kidney Cancer [, , , , , , , , , , ]
Q9: Has this compound shown efficacy in in vivo models?
A9: Yes, this compound and its analogs (KPT-251, KPT-276, KPT-330) have demonstrated significant antitumor activity in various in vivo models, including xenograft models of MCL, AML, MM, NSCLC, pancreatic cancer, ovarian cancer, and melanoma. These studies highlight the potential of this compound as a therapeutic agent. [, , , , , , , , , , , ]
Q10: Are there any clinical trials investigating this compound?
A10: The research mentions that KPT-330 (selinexor), a clinically relevant analog of this compound, has entered Phase I clinical trials for hematological malignancies and solid tumors. [, , , ]
Q11: What are the potential mechanisms of resistance to this compound?
A11: Although not extensively discussed, research suggests that mutations in the CRM1 binding site for SINE compounds, particularly at Cys-528, can confer resistance. Additionally, alterations in downstream pathways or compensatory mechanisms that bypass CRM1 function could also contribute to resistance. [, ]
Q12: Are there any known biomarkers to predict the efficacy of this compound?
A12: While specific biomarkers for this compound are not extensively discussed, the research suggests that CRM1 expression levels could be a potential predictive biomarker. Tumors with higher CRM1 expression might exhibit increased sensitivity to this compound treatment. [, ] Further research is needed to validate this hypothesis and identify additional biomarkers.
Q13: What analytical techniques are used to characterize and quantify this compound?
A13: The provided research papers employ various standard analytical techniques to investigate this compound and its effects, including:
- Cell viability assays: MTT assay, trypan blue exclusion assay [, , , , , , , , , , , , , , , , ]
- Apoptosis assays: Annexin V/PI staining, Cell Death Detection ELISA [, , , , , , , , , , , , , , ]
- Cell cycle analysis: Flow cytometry with propidium iodide staining []
- Protein analysis: Western blotting, immunofluorescence microscopy, co-immunoprecipitation [, , , , , , , , , , , , , , , , , , , , , ]
- Gene expression analysis: TaqMan Low Density Arrays, microarray analysis [, , , , ]
- Proteomics: Isobaric Tags for Relative and Absolute Quantification (iTRAQ) [, , ]
- Metabolite analysis: Capillary Electrophoresis Mass Spectrometry (CETOF-MS) []
- In vivo imaging: Whole Body Luminescence Imaging (WBLI) []
Disclaimer and Information on In-Vitro Research Products
Please be aware that all articles and product information presented on BenchChem are intended solely for informational purposes. The products available for purchase on BenchChem are specifically designed for in-vitro studies, which are conducted outside of living organisms. In-vitro studies, derived from the Latin term "in glass," involve experiments performed in controlled laboratory settings using cells or tissues. It is important to note that these products are not categorized as medicines or drugs, and they have not received approval from the FDA for the prevention, treatment, or cure of any medical condition, ailment, or disease. We must emphasize that any form of bodily introduction of these products into humans or animals is strictly prohibited by law. It is essential to adhere to these guidelines to ensure compliance with legal and ethical standards in research and experimentation.