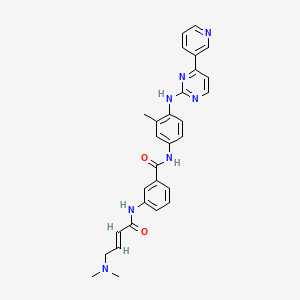
Jnk-IN-8
Overview
Description
JNK-IN-8 is a potent and selective inhibitor of c-Jun N-terminal kinases (JNKs), which are part of the mitogen-activated protein kinase (MAPK) family. JNKs play a crucial role in regulating various physiological processes, including cell proliferation, differentiation, survival, and apoptosis. Dysregulated JNK signaling has been implicated in several diseases, including cancer, making this compound a valuable tool in scientific research and potential therapeutic applications .
Mechanism of Action
Target of Action
Jnk-IN-8 is an irreversible inhibitor of c-Jun N-terminal kinases (JNKs), specifically JNK1, JNK2, and JNK3 . JNKs are members of the mitogen-activated protein kinases (MAPK) family, which regulate many physiological processes including inflammatory responses, cell proliferation, differentiation, survival, and death .
Mode of Action
This compound inhibits JNK signaling by inhibiting the phosphorylation of the JNK substrate, c-Jun . This inhibition is highly specific to JNKs . The compound forms a covalent bond with a conserved cysteine residue in the activation loop of JNKs, leading to a conformational change that prevents substrate binding .
Biochemical Pathways
The JNK pathway is activated in response to various stimuli such as inflammation, oxidative stress, infection, osmotic stress, DNA damage, or cytoskeletal changes . This compound’s inhibition of JNK signaling affects these pathways and their downstream effects. For instance, this compound has been shown to activate TFEB and TFE3, master regulators of lysosome biogenesis and autophagy, via mTOR inhibition independently of JNK .
Result of Action
This compound has been shown to suppress tumor growth in various cancer types, such as triple-negative breast cancer (TNBC) and pancreatic ductal adenocarcinoma (PDAC), both in vitro and in vivo . It reduces colony formation, cell viability, and organoid growth in vitro and slows patient-derived xenograft and syngeneic tumor growth in vivo . Moreover, this compound has been reported to induce lysosome biogenesis and autophagy by activating TFEB/TFE3 .
Biochemical Analysis
Biochemical Properties
Jnk-IN-8 inhibits phosphorylation of c-Jun . It interacts with JNK1, JNK2, and JNK3 . The activation of JNK requires dual phosphorylation at its Try and Thr residues, which is mediated by MKK4 and MKK7 .
Cellular Effects
This compound has been shown to suppress tumor growth in triple-negative breast cancer by activating TFEB- and TFE3-mediated lysosome biogenesis and autophagy . It reduces colony formation, cell viability, and organoid growth in vitro .
Molecular Mechanism
This compound inhibits TFEB phosphorylation and induces nuclear translocation of unphosphorylated TFEB and TFE3 . This is accompanied by an upregulation of TFEB/TFE3 target genes associated with lysosome biogenesis and autophagy .
Preparation Methods
Synthetic Routes and Reaction Conditions: JNK-IN-8 is synthesized through a multi-step chemical process. The synthesis involves the formation of a covalent bond with the conserved cysteine residue of JNK1, JNK2, and JNK3. The key steps include:
- Formation of the core structure through a series of condensation and cyclization reactions.
- Introduction of functional groups to enhance specificity and potency.
- Purification and characterization of the final product using techniques such as high-performance liquid chromatography (HPLC) and mass spectrometry .
Industrial Production Methods: While specific industrial production methods for this compound are not widely documented, the general approach involves scaling up the laboratory synthesis process. This includes optimizing reaction conditions, using industrial-grade reagents, and employing large-scale purification techniques to ensure high yield and purity .
Chemical Reactions Analysis
Types of Reactions: JNK-IN-8 primarily undergoes covalent bonding reactions with its target kinases. It forms a covalent bond with the conserved cysteine residue in the activation loop of JNK1, JNK2, and JNK3, leading to irreversible inhibition .
Common Reagents and Conditions:
Reagents: The synthesis of this compound involves reagents such as organic solvents, catalysts, and protective groups.
Conditions: The reactions are typically carried out under controlled temperature and pH conditions to ensure specificity and yield
Major Products: The major product of the reaction is the covalently bonded this compound-kinase complex, which results in the inhibition of JNK signaling pathways .
Scientific Research Applications
Comparison with Similar Compounds
SP600125: A reversible JNK inhibitor that competes with ATP for binding to the kinase domain.
AS601245: Another JNK inhibitor with a different chemical structure.
Uniqueness of JNK-IN-8: this compound is unique due to its irreversible inhibition mechanism and high specificity for JNK1, JNK2, and JNK3. This specificity reduces off-target effects and enhances its potential as a therapeutic agent .
Properties
IUPAC Name |
3-[[(E)-4-(dimethylamino)but-2-enoyl]amino]-N-[3-methyl-4-[(4-pyridin-3-ylpyrimidin-2-yl)amino]phenyl]benzamide | |
---|---|---|
Source | PubChem | |
URL | https://pubchem.ncbi.nlm.nih.gov | |
Description | Data deposited in or computed by PubChem | |
InChI |
InChI=1S/C29H29N7O2/c1-20-17-24(11-12-25(20)34-29-31-15-13-26(35-29)22-8-5-14-30-19-22)33-28(38)21-7-4-9-23(18-21)32-27(37)10-6-16-36(2)3/h4-15,17-19H,16H2,1-3H3,(H,32,37)(H,33,38)(H,31,34,35)/b10-6+ | |
Source | PubChem | |
URL | https://pubchem.ncbi.nlm.nih.gov | |
Description | Data deposited in or computed by PubChem | |
InChI Key |
GJFCSAPFHAXMSF-UXBLZVDNSA-N | |
Source | PubChem | |
URL | https://pubchem.ncbi.nlm.nih.gov | |
Description | Data deposited in or computed by PubChem | |
Canonical SMILES |
CC1=C(C=CC(=C1)NC(=O)C2=CC(=CC=C2)NC(=O)C=CCN(C)C)NC3=NC=CC(=N3)C4=CN=CC=C4 | |
Source | PubChem | |
URL | https://pubchem.ncbi.nlm.nih.gov | |
Description | Data deposited in or computed by PubChem | |
Isomeric SMILES |
CC1=C(C=CC(=C1)NC(=O)C2=CC(=CC=C2)NC(=O)/C=C/CN(C)C)NC3=NC=CC(=N3)C4=CN=CC=C4 | |
Source | PubChem | |
URL | https://pubchem.ncbi.nlm.nih.gov | |
Description | Data deposited in or computed by PubChem | |
Molecular Formula |
C29H29N7O2 | |
Source | PubChem | |
URL | https://pubchem.ncbi.nlm.nih.gov | |
Description | Data deposited in or computed by PubChem | |
DSSTOX Substance ID |
DTXSID40720937 | |
Record name | 3-{[(2E)-4-(Dimethylamino)but-2-enoyl]amino}-N-(3-methyl-4-{[4-(pyridin-3-yl)pyrimidin-2-yl]amino}phenyl)benzamide | |
Source | EPA DSSTox | |
URL | https://comptox.epa.gov/dashboard/DTXSID40720937 | |
Description | DSSTox provides a high quality public chemistry resource for supporting improved predictive toxicology. | |
Molecular Weight |
507.6 g/mol | |
Source | PubChem | |
URL | https://pubchem.ncbi.nlm.nih.gov | |
Description | Data deposited in or computed by PubChem | |
CAS No. |
1410880-22-6 | |
Record name | 3-{[(2E)-4-(Dimethylamino)but-2-enoyl]amino}-N-(3-methyl-4-{[4-(pyridin-3-yl)pyrimidin-2-yl]amino}phenyl)benzamide | |
Source | EPA DSSTox | |
URL | https://comptox.epa.gov/dashboard/DTXSID40720937 | |
Description | DSSTox provides a high quality public chemistry resource for supporting improved predictive toxicology. | |
Q1: How does JNK-IN-8 interact with its target, JNK?
A: this compound is an ATP-competitive inhibitor that forms a covalent bond with a conserved cysteine residue (Cys116) located within the ATP-binding pocket of JNK. [, , , , , , , , ] This irreversible binding distinguishes it from reversible JNK inhibitors like SP600125, which demonstrates lower selectivity for JNK. [, ]
Q2: What are the downstream consequences of this compound-mediated JNK inhibition?
A2: JNK inhibition by this compound leads to various downstream effects depending on the cell type and context. These include:
- Reduced Cell Proliferation and Increased Apoptosis: Observed in various cancer cell lines, including triple-negative breast cancer (TNBC), osteosarcoma, and melanoma. [, , , , , , , , , , , , , , , , ]
- Activation of Lysosome Biogenesis and Autophagy: this compound induces lysosome biogenesis and autophagy in TNBC cells by inhibiting mTOR signaling and activating the transcription factors TFEB and TFE3. [, ]
- Suppression of Inflammation and Oxidative Stress: this compound reduces inflammatory cytokine production and oxidative stress in models of acute lung injury and ischemic stroke. [, ]
- Modulation of Immune Cell Infiltration: Treatment with this compound reduced tumor-infiltrating regulatory T cells (Tregs) and myeloid-derived suppressor cells (MDSCs), while increasing CD8+ T cell infiltration in a TNBC mouse model. [] This suggests potential for enhancing immune checkpoint inhibitor efficacy.
Q3: Does this compound inhibit all JNK isoforms equally?
A: While this compound can bind to all three JNK genes (JNK1, JNK2, and JNK3), studies suggest distinct roles for each isoform in certain cancers, implying potential benefit for isoform-selective inhibitors. [, ] For example, JNK1 appears crucial in UV-induced apoptosis in skin cancer, while JNK2 promotes melanoma cell proliferation and invasiveness. [, ]
Q4: What is the molecular formula and weight of this compound?
A4: Unfortunately, the provided research abstracts do not disclose the molecular formula, weight, or spectroscopic data for this compound.
Q5: What is known about the stability of this compound under various conditions?
A5: The research abstracts provide limited information regarding this compound's stability under different conditions. Further research is necessary to determine its stability profile and develop appropriate formulation strategies for improved stability, solubility, and bioavailability.
Q6: What is known about the pharmacokinetics and pharmacodynamics of this compound?
A: Although specific details regarding absorption, distribution, metabolism, and excretion (ADME) are limited within the provided research, this compound demonstrates in vivo efficacy in preclinical models. It significantly inhibited tumor growth in TNBC xenograft models [, ] and prolonged survival in mice with BCR-ABL-induced B-ALL when combined with dasatinib. [] It also alleviated lipopolysaccharide-induced acute lung injury and improved functional recovery in ischemic stroke models. [, ]
Q7: Are there any known resistance mechanisms to this compound?
A: One study identified that bypass of this compound-mediated JNK inhibition by expressing a JNK2 mutant (JNK2C116S) led to enhanced tumor growth in a melanoma model, suggesting a potential resistance mechanism. [] This highlights the need for understanding resistance mechanisms and exploring combination therapies.
Q8: Have any biomarkers been identified for predicting this compound efficacy or monitoring treatment response?
A: One study identified a JNK-active TNBC cluster associated with an immunosuppressive TME, suggesting that JNK pathway activation and associated biomarkers could predict response to JNK-targeted therapy. []
Disclaimer and Information on In-Vitro Research Products
Please be aware that all articles and product information presented on BenchChem are intended solely for informational purposes. The products available for purchase on BenchChem are specifically designed for in-vitro studies, which are conducted outside of living organisms. In-vitro studies, derived from the Latin term "in glass," involve experiments performed in controlled laboratory settings using cells or tissues. It is important to note that these products are not categorized as medicines or drugs, and they have not received approval from the FDA for the prevention, treatment, or cure of any medical condition, ailment, or disease. We must emphasize that any form of bodily introduction of these products into humans or animals is strictly prohibited by law. It is essential to adhere to these guidelines to ensure compliance with legal and ethical standards in research and experimentation.