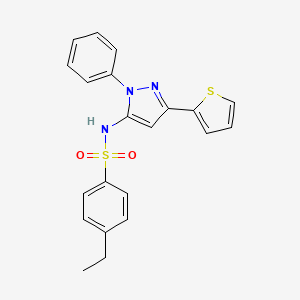
HSF1A
Overview
Description
HSF1A, also known as Heat Shock Factor 1 Activator, is a small molecule compound that activates heat shock transcription factor 1 (HSF1). HSF1 is a transcription factor that plays a crucial role in the cellular response to proteotoxic stress, such as heat shock and oxidative stress. This compound has been identified as a potential therapeutic agent for neurodegenerative diseases and other conditions associated with protein misfolding .
Mechanism of Action
Target of Action
HSF1A, also known as “4-Ethyl-N-(1-phenyl-3-(thiophen-2-yl)-1H-pyrazol-5-yl)benzenesulfonamide” or “4-Ethyl-N-[1-phenyl-3-(2-thienyl)-1H-pyrazol-5-yl]-benzenesulfonamide”, primarily targets the Heat Shock Transcription Factor 1 (HSF1) . HSF1 is the master transcriptional regulator of the heat shock response (HSR) in mammalian cells and plays a critical role in maintaining protein homeostasis . It is involved in many physiological processes like embryogenesis, metabolism, immune response, aging, cancer, and neurodegeneration .
Mode of Action
This compound is a cell-permeable activator of HSF1 . It activates HSF1 in mammalian and fly cells, elevates protein chaperone expression, and ameliorates protein misfolding . This compound also interacts with components of the TRiC/CCT complex, suggesting a potentially novel regulatory role for this complex in modulating HSF1 activity .
Biochemical Pathways
This compound affects the heat shock response pathway by activating HSF1 . This leads to the transcriptional activation of genes encoding protein chaperones in response to proteotoxic conditions . These protein chaperones assist in the correct folding of other proteins, suppressing the neurotoxicity of misfolded proteins .
Pharmacokinetics
It is known to be cell-permeable , suggesting that it can cross cell membranes to exert its effects.
Result of Action
This compound’s activation of HSF1 leads to elevated protein chaperone expression, which can suppress protein misfolding and cell death in polyQ-expressing neuronal precursor cells . It also protects against cytotoxicity in a fly model of polyQ-mediated neurodegeneration . In the context of pertussis toxin (PT) intoxication, this compound effectively reduces the levels of ADP-ribosylated Gαi, the specific substrate of PT, in PT-treated cells .
Action Environment
The action of this compound can be influenced by environmental factors such as heat shock conditions . For example, in oysters, different heat shock conditions led to various combinations of HSF1 isoforms, which could potentially influence the efficacy of this compound .
Biochemical Analysis
Biochemical Properties
HSF1A plays a pivotal role in biochemical reactions by activating HSF1, a master regulator of the heat shock response. This activation leads to the increased expression of heat shock proteins (HSPs), which function as molecular chaperones to assist in the proper folding of proteins and the prevention of protein aggregation. This compound interacts with components of the TRiC/CCT complex, suggesting a novel regulatory role for this complex in modulating HSF1 activity . The compound’s interaction with HSF1 is crucial for its ability to enhance the cellular stress response, thereby mitigating the effects of protein misfolding.
Cellular Effects
This compound exerts significant effects on various cell types and cellular processes. By activating HSF1, this compound induces the expression of HSPs, which play a critical role in maintaining cellular proteostasis. This activation influences cell signaling pathways, gene expression, and cellular metabolism. For instance, in neuronal precursor cells, this compound has been shown to ameliorate protein misfolding and cell death, highlighting its potential in treating neurodegenerative conditions . Additionally, this compound’s impact on cell signaling pathways can lead to enhanced cellular resilience against stress-induced damage.
Molecular Mechanism
The molecular mechanism of this compound involves its binding to HSF1, leading to the activation of this transcription factor. Upon activation, HSF1 trimerizes and binds to heat shock elements (HSEs) in the promoter regions of target genes, initiating the transcription of HSPs. This process is crucial for the cellular stress response, as it enhances the cell’s ability to cope with proteotoxic stress. This compound’s interaction with the TRiC/CCT complex further modulates HSF1 activity, suggesting a complex regulatory network that ensures precise control of the heat shock response .
Temporal Effects in Laboratory Settings
In laboratory settings, the effects of this compound have been observed to change over time. The compound’s stability and degradation are critical factors influencing its long-term efficacy. Studies have shown that this compound remains stable under specific storage conditions, but its activity can diminish over extended periods due to degradation . Long-term exposure to this compound in in vitro and in vivo studies has demonstrated sustained activation of HSF1 and increased expression of HSPs, indicating its potential for prolonged therapeutic use.
Dosage Effects in Animal Models
The effects of this compound vary with different dosages in animal models. At optimal doses, this compound effectively activates HSF1 and induces the expression of HSPs, leading to improved cellular resilience against stress. At higher doses, this compound may exhibit toxic or adverse effects, including cytotoxicity and disruption of normal cellular functions . Determining the appropriate dosage is crucial for maximizing the therapeutic benefits of this compound while minimizing potential side effects.
Metabolic Pathways
This compound is involved in metabolic pathways that regulate protein homeostasis. By activating HSF1, this compound enhances the expression of HSPs, which are essential for protein folding and degradation. This activation influences metabolic flux and metabolite levels, ensuring the proper functioning of cellular processes under stress conditions . The interaction of this compound with enzymes and cofactors involved in these pathways underscores its role in maintaining cellular proteostasis.
Transport and Distribution
Within cells and tissues, this compound is transported and distributed through various mechanisms. The compound’s cell-permeable nature allows it to efficiently reach its target sites, including the nucleus where HSF1 resides . Transporters and binding proteins may facilitate the localization and accumulation of this compound, ensuring its effective interaction with HSF1 and subsequent activation of the heat shock response.
Subcellular Localization
This compound’s subcellular localization is primarily within the nucleus, where it interacts with HSF1 to initiate the transcription of HSPs. The compound’s targeting signals and potential post-translational modifications may direct it to specific nuclear compartments, enhancing its activity and function . This precise localization is essential for the effective modulation of the heat shock response and the maintenance of cellular proteostasis.
Preparation Methods
Synthetic Routes and Reaction Conditions
HSF1A is a benzyl pyrazole derivative. The synthesis of this compound involves the reaction of benzyl bromide with pyrazole in the presence of a base, such as potassium carbonate, in an organic solvent like dimethyl sulfoxide (DMSO). The reaction is typically carried out at elevated temperatures to facilitate the formation of the desired product .
Industrial Production Methods
Industrial production of this compound follows similar synthetic routes but on a larger scale. The process involves optimizing reaction conditions to maximize yield and purity. High-throughput screening methods are often employed to identify the most efficient synthetic pathways and reaction conditions .
Chemical Reactions Analysis
Types of Reactions
HSF1A undergoes various chemical reactions, including:
Oxidation: this compound can be oxidized to form corresponding sulfoxides and sulfones.
Reduction: Reduction of this compound can lead to the formation of its corresponding amines.
Substitution: This compound can undergo nucleophilic substitution reactions, where the benzyl group can be replaced by other nucleophiles.
Common Reagents and Conditions
Oxidation: Common oxidizing agents include hydrogen peroxide and m-chloroperbenzoic acid.
Reduction: Reducing agents such as lithium aluminum hydride and sodium borohydride are used.
Substitution: Nucleophiles like amines, thiols, and alcohols can be used in substitution reactions.
Major Products Formed
Oxidation: Formation of sulfoxides and sulfones.
Reduction: Formation of amines.
Substitution: Formation of substituted benzyl derivatives.
Scientific Research Applications
HSF1A has several scientific research applications, including:
Neurodegenerative Diseases: this compound has been shown to activate HSF1, leading to the expression of molecular chaperones that suppress protein misfolding. .
Cancer Research: this compound has been investigated for its role in cancer therapy.
Cellular Stress Response: This compound is used to study the cellular stress response mechanisms, particularly the role of HSF1 in regulating the expression of heat shock proteins.
Comparison with Similar Compounds
HSF1A is unique compared to other HSF1 activators due to its specific interaction with the TRiC/CCT complex. Similar compounds include:
KRIBB11: Another HSF1 activator that inhibits heat shock protein 90 (Hsp90) and induces HSF1 activity.
Bis-(2-hydroxybenzylidine) acetone: A synthetic derivative that induces HSF1 activity through inhibition of heat shock protein 90 (Hsp90).
This compound’s specificity for the TRiC/CCT complex and its ability to activate HSF1 without perturbing ATP hydrolysis make it a unique and valuable compound for scientific research .
Biological Activity
Heat Shock Factor 1 (HSF1) is a critical transcription factor involved in the cellular response to stress, particularly in the regulation of heat shock proteins (HSPs). This article explores the biological activity of HSF1, focusing on its mechanisms, implications in cancer, neuroprotection, and its role in inflammation.
Mechanisms of HSF1 Activation
HSF1 is primarily activated in response to proteotoxic stress, such as heat shock. Upon activation, HSF1 undergoes a conformational change from a monomer to a trimer, which allows it to translocate into the nucleus and bind to heat shock elements (HSEs) in the promoter regions of target genes. This process is essential for the transcriptional regulation of HSPs, which play a vital role in protein folding and protection against cellular stress.
Key Findings:
- Trimerization : HSF1's transition to a trimeric form is crucial for its DNA binding and transcriptional activity .
- Transcriptional Regulation : HSF1 recruits various transcriptional and DNA-modifying proteins to regulate gene expression during stress responses .
Role in Cancer
HSF1 has been implicated in tumorigenesis and cancer progression. Studies have shown that HSF1 is frequently activated in various cancer types, including breast, colon, and lung cancers. Its activation correlates with poor prognosis and enhanced metastatic potential.
Case Studies:
- In breast cancer models, HSF1 was found to inhibit antitumor immune activity by reducing CCL5 expression, thereby limiting CD8+ T-cell homing to tumors .
- A study demonstrated that HSF1 regulates a distinct transcriptional program associated with malignancy, influencing genes related to metastasis and cell survival .
Neuroprotection
HSF1 also plays a significant role in neuroprotection. Its activation has been linked to the enhancement of cellular resilience against apoptosis and neurodegenerative conditions.
Research Findings:
- In cerebellar granule neurons subjected to apoptotic conditions, overexpression of HSF1 resulted in altered expression of numerous genes associated with neuroprotection. The study identified 1,266 differentially expressed genes (DEGs) regulated by HSF1 under varying potassium conditions .
- The protective effects of HSF1 are thought to stem from its ability to enhance the expression of genes involved in cellular stress responses.
Inflammation Modulation
Recent research indicates that HSF1 can modulate inflammatory responses following heat shock. It inhibits excessive activation of inflammatory genes such as ATF3 and JUN&FOS, suggesting a protective role against inflammation induced by stress .
Data Tables
Here are key findings summarized in tables for clarity:
Properties
IUPAC Name |
4-ethyl-N-(2-phenyl-5-thiophen-2-ylpyrazol-3-yl)benzenesulfonamide | |
---|---|---|
Source | PubChem | |
URL | https://pubchem.ncbi.nlm.nih.gov | |
Description | Data deposited in or computed by PubChem | |
InChI |
InChI=1S/C21H19N3O2S2/c1-2-16-10-12-18(13-11-16)28(25,26)23-21-15-19(20-9-6-14-27-20)22-24(21)17-7-4-3-5-8-17/h3-15,23H,2H2,1H3 | |
Source | PubChem | |
URL | https://pubchem.ncbi.nlm.nih.gov | |
Description | Data deposited in or computed by PubChem | |
InChI Key |
KJTITGSAONQVPY-UHFFFAOYSA-N | |
Source | PubChem | |
URL | https://pubchem.ncbi.nlm.nih.gov | |
Description | Data deposited in or computed by PubChem | |
Canonical SMILES |
CCC1=CC=C(C=C1)S(=O)(=O)NC2=CC(=NN2C3=CC=CC=C3)C4=CC=CS4 | |
Source | PubChem | |
URL | https://pubchem.ncbi.nlm.nih.gov | |
Description | Data deposited in or computed by PubChem | |
Molecular Formula |
C21H19N3O2S2 | |
Source | PubChem | |
URL | https://pubchem.ncbi.nlm.nih.gov | |
Description | Data deposited in or computed by PubChem | |
Molecular Weight |
409.5 g/mol | |
Source | PubChem | |
URL | https://pubchem.ncbi.nlm.nih.gov | |
Description | Data deposited in or computed by PubChem | |
Retrosynthesis Analysis
AI-Powered Synthesis Planning: Our tool employs the Template_relevance Pistachio, Template_relevance Bkms_metabolic, Template_relevance Pistachio_ringbreaker, Template_relevance Reaxys, Template_relevance Reaxys_biocatalysis model, leveraging a vast database of chemical reactions to predict feasible synthetic routes.
One-Step Synthesis Focus: Specifically designed for one-step synthesis, it provides concise and direct routes for your target compounds, streamlining the synthesis process.
Accurate Predictions: Utilizing the extensive PISTACHIO, BKMS_METABOLIC, PISTACHIO_RINGBREAKER, REAXYS, REAXYS_BIOCATALYSIS database, our tool offers high-accuracy predictions, reflecting the latest in chemical research and data.
Strategy Settings
Precursor scoring | Relevance Heuristic |
---|---|
Min. plausibility | 0.01 |
Model | Template_relevance |
Template Set | Pistachio/Bkms_metabolic/Pistachio_ringbreaker/Reaxys/Reaxys_biocatalysis |
Top-N result to add to graph | 6 |
Feasible Synthetic Routes
Disclaimer and Information on In-Vitro Research Products
Please be aware that all articles and product information presented on BenchChem are intended solely for informational purposes. The products available for purchase on BenchChem are specifically designed for in-vitro studies, which are conducted outside of living organisms. In-vitro studies, derived from the Latin term "in glass," involve experiments performed in controlled laboratory settings using cells or tissues. It is important to note that these products are not categorized as medicines or drugs, and they have not received approval from the FDA for the prevention, treatment, or cure of any medical condition, ailment, or disease. We must emphasize that any form of bodily introduction of these products into humans or animals is strictly prohibited by law. It is essential to adhere to these guidelines to ensure compliance with legal and ethical standards in research and experimentation.