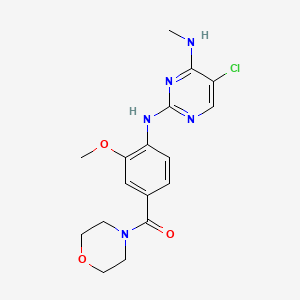
Hg-10-102-01
- Click on QUICK INQUIRY to receive a quote from our team of experts.
- With the quality product at a COMPETITIVE price, you can focus more on your research.
Overview
Description
HG-10-102-01 is a highly potent, selective, and brain-penetrable inhibitor of leucine-rich repeat kinase 2 (LRRK2). It has shown significant efficacy in inhibiting both wild-type LRRK2 and its mutant forms, particularly the G2019S mutation, which is commonly associated with Parkinson’s disease . The compound also inhibits mitogen-activated protein kinase-interacting kinase 2 (MNK2) and mixed-lineage kinase 1 (MLK1) .
Mechanism of Action
Target of Action
HG-10-102-01 is a highly potent, selective, and brain-penetrable inhibitor . Its primary targets are Leucine-rich repeat kinase 2 (LRRK2) , MNK2 , and MLK1 . LRRK2 is a protein that is associated with Parkinson’s disease . MNK2 and MLK1 are kinases involved in various cellular processes .
Mode of Action
This compound acts as an ATP-competitive inhibitor . It inhibits the kinase activities of its targets by competing with ATP for binding to the ATP-binding site . The IC50 values against wild-type LRRK2 and LRRK2 [G2019S] are 20.3 nM and 3.2 nM respectively . It also inhibits MNK2 and MLK1 with IC50 values of 0.6 μM and 2.1 μM respectively .
Biochemical Pathways
It is known that lrrk2, one of the primary targets of this compound, plays a role in several cellular processes, including vesicle trafficking, autophagy, and cytoskeletal dynamics . By inhibiting LRRK2, this compound could potentially affect these processes.
Pharmacokinetics
It is noted that this compound is brain-penetrable , suggesting that it can cross the blood-brain barrier, which is crucial for its potential use in treating neurological disorders like Parkinson’s disease .
Result of Action
This compound substantially inhibits Ser910 and Ser935 phosphorylation of both wild-type LRRK2 and G2019S mutant . This suggests that this compound can modulate the phosphorylation state of LRRK2, which may have downstream effects on LRRK2’s function and the cellular processes it is involved in .
Action Environment
Like all drugs, the action of this compound could potentially be influenced by various factors, including the physiological state of the patient, the presence of other drugs, and specific characteristics of the disease state .
Biochemical Analysis
Biochemical Properties
Hg-10-102-01 plays a crucial role in biochemical reactions by inhibiting LRRK2, a kinase involved in various cellular processes. The compound interacts with enzymes such as mitogen-activated protein kinase-interacting kinase 2 (MNK2) and mixed lineage kinase 1 (MLK1), with IC50 values of 0.6 μM and 2.1 μM, respectively . These interactions result in the inhibition of phosphorylation at specific serine residues (Ser910 and Ser935) in LRRK2, which is essential for its activity .
Cellular Effects
This compound affects various types of cells, including HEK293 cells, mouse Swiss 3T3 cells, and mouse embryonic fibroblast cells. The compound induces a dose-dependent inhibition of Ser910 and Ser935 phosphorylation in both wild-type LRRK2 and the G2019S mutant . This inhibition impacts cell signaling pathways, gene expression, and cellular metabolism, leading to altered cellular functions .
Molecular Mechanism
The molecular mechanism of this compound involves its binding to the active site of LRRK2, thereby inhibiting its kinase activity. This inhibition prevents the phosphorylation of downstream targets, such as Ser910 and Ser935, which are critical for LRRK2’s function . Additionally, this compound inhibits MNK2 and MLK1, further affecting cellular signaling pathways .
Temporal Effects in Laboratory Settings
In laboratory settings, this compound demonstrates stability and sustained inhibitory effects over time. In vitro studies show that the compound can inhibit LRRK2 phosphorylation for up to 90 minutes at concentrations ranging from 0.01 to 3 μM . In vivo studies indicate that a single intraperitoneal injection of this compound can inhibit LRRK2 phosphorylation in the kidney, spleen, and brain of mice .
Dosage Effects in Animal Models
The effects of this compound vary with different dosages in animal models. In wild-type male C57BL/6 mice, doses ranging from 3 to 100 mg/kg administered intraperitoneally result in dose-dependent inhibition of LRRK2 phosphorylation . Higher doses may lead to toxic or adverse effects, highlighting the importance of determining the optimal dosage for therapeutic applications .
Metabolic Pathways
This compound is involved in metabolic pathways related to LRRK2 activity. The compound interacts with enzymes and cofactors that regulate phosphorylation and dephosphorylation processes. This interaction affects metabolic flux and metabolite levels, contributing to its overall biochemical effects .
Transport and Distribution
This compound is transported and distributed within cells and tissues through specific transporters and binding proteins. The compound’s brain-penetrable nature allows it to accumulate in the brain, where it exerts its inhibitory effects on LRRK2 . This distribution is crucial for its potential therapeutic applications in neurodegenerative diseases .
Subcellular Localization
The subcellular localization of this compound is primarily within the cytoplasm, where it interacts with LRRK2 and other target proteins. The compound’s activity is influenced by its localization, as it needs to be in proximity to its targets to exert its inhibitory effects . Post-translational modifications and targeting signals may also play a role in directing this compound to specific cellular compartments .
Preparation Methods
The synthesis of HG-10-102-01 involves multiple steps, starting with the preparation of the core structure, followed by functional group modifications to enhance its potency and selectivity. The synthetic route typically includes the following steps:
Formation of the Core Structure: The core structure is synthesized using a series of condensation reactions involving aromatic amines and chloropyrimidines.
Functional Group Modifications: The core structure is then modified by introducing various functional groups to enhance its inhibitory activity and brain penetration capabilities.
Purification: The final product is purified using techniques such as recrystallization and chromatography to achieve high purity levels
Industrial production methods for this compound are not widely documented, but they likely involve scaling up the laboratory synthesis process with optimizations for yield and cost-effectiveness.
Chemical Reactions Analysis
HG-10-102-01 undergoes several types of chemical reactions, including:
Oxidation: The compound can undergo oxidation reactions, particularly at the methoxy and amino groups, leading to the formation of various oxidized derivatives.
Reduction: Reduction reactions can occur at the nitro and carbonyl groups, resulting in the formation of reduced derivatives.
Substitution: The compound can undergo nucleophilic substitution reactions, particularly at the chloro and amino groups, leading to the formation of substituted derivatives
Common reagents and conditions used in these reactions include oxidizing agents like potassium permanganate, reducing agents like sodium borohydride, and nucleophiles like amines and thiols. The major products formed from these reactions are typically derivatives of this compound with modified functional groups.
Scientific Research Applications
HG-10-102-01 has a wide range of scientific research applications, including:
Biology: this compound is used to study the biological functions of LRRK2 and its role in cellular signaling pathways. .
Medicine: The compound is primarily used in Parkinson’s disease research to study the effects of LRRK2 inhibition on disease progression and to develop new therapeutic strategies for treating the disease
Comparison with Similar Compounds
HG-10-102-01 is unique in its high potency, selectivity, and brain penetration capabilities compared to other LRRK2 inhibitors. Similar compounds include:
LRRK2-IN-1: Another potent LRRK2 inhibitor, but with lower brain penetration capabilities compared to this compound.
This compound stands out due to its ability to effectively inhibit both wild-type and mutant forms of LRRK2, particularly the G2019S mutation, and its superior brain penetration capabilities .
Properties
IUPAC Name |
[4-[[5-chloro-4-(methylamino)pyrimidin-2-yl]amino]-3-methoxyphenyl]-morpholin-4-ylmethanone |
Source
|
---|---|---|
Source | PubChem | |
URL | https://pubchem.ncbi.nlm.nih.gov | |
Description | Data deposited in or computed by PubChem | |
InChI |
InChI=1S/C17H20ClN5O3/c1-19-15-12(18)10-20-17(22-15)21-13-4-3-11(9-14(13)25-2)16(24)23-5-7-26-8-6-23/h3-4,9-10H,5-8H2,1-2H3,(H2,19,20,21,22) |
Source
|
Source | PubChem | |
URL | https://pubchem.ncbi.nlm.nih.gov | |
Description | Data deposited in or computed by PubChem | |
InChI Key |
YEVOZZZLKJKCCD-UHFFFAOYSA-N |
Source
|
Source | PubChem | |
URL | https://pubchem.ncbi.nlm.nih.gov | |
Description | Data deposited in or computed by PubChem | |
Canonical SMILES |
CNC1=NC(=NC=C1Cl)NC2=C(C=C(C=C2)C(=O)N3CCOCC3)OC |
Source
|
Source | PubChem | |
URL | https://pubchem.ncbi.nlm.nih.gov | |
Description | Data deposited in or computed by PubChem | |
Molecular Formula |
C17H20ClN5O3 |
Source
|
Source | PubChem | |
URL | https://pubchem.ncbi.nlm.nih.gov | |
Description | Data deposited in or computed by PubChem | |
Molecular Weight |
377.8 g/mol |
Source
|
Source | PubChem | |
URL | https://pubchem.ncbi.nlm.nih.gov | |
Description | Data deposited in or computed by PubChem | |
Q1: How does HG-10-102-01 interact with its target and what are the downstream effects?
A: this compound acts as a potent and selective inhibitor of leucine-rich repeat kinase 2 (LRRK2), both in its wild-type form and the G2019S mutant form. [] This kinase is implicated in the pathogenesis of Parkinson's disease (PD). The compound effectively inhibits the phosphorylation of Ser910 and Ser935 residues on LRRK2, even at low micromolar concentrations. [] This inhibition is significant as elevated LRRK2 activity is linked to PD development.
Q2: What is known about the structure-activity relationship (SAR) of this compound and how do modifications impact its properties?
A: Researchers have explored modifications to the this compound structure to enhance its properties, particularly its ability to cross the blood-brain barrier (BBB), a major obstacle for many CNS drugs. [] By linking the terminal nitrogen atoms of this compound with alkyl chains of varying lengths, they created macrocyclic molecules. [] This modification, guided by 3D-QSAR models, resulted in several derivatives with improved BBB permeability while retaining nanomolar inhibitory potency against LRRK2. []
Q3: What in vitro and in vivo studies have been conducted on this compound?
A: this compound has demonstrated potent inhibition of LRRK2 phosphorylation in cellular assays. [] Furthermore, it effectively inhibits LRRK2 activity in vivo. Notably, it can inhibit the phosphorylation of Ser910 and Ser935 in the mouse brain following intraperitoneal administration. [] This brain penetrance makes it a promising candidate for further development as a PD therapeutic. Further research, including detailed pharmacological profiling and assessments of its effectiveness in relevant PD models, are ongoing. []
Disclaimer and Information on In-Vitro Research Products
Please be aware that all articles and product information presented on BenchChem are intended solely for informational purposes. The products available for purchase on BenchChem are specifically designed for in-vitro studies, which are conducted outside of living organisms. In-vitro studies, derived from the Latin term "in glass," involve experiments performed in controlled laboratory settings using cells or tissues. It is important to note that these products are not categorized as medicines or drugs, and they have not received approval from the FDA for the prevention, treatment, or cure of any medical condition, ailment, or disease. We must emphasize that any form of bodily introduction of these products into humans or animals is strictly prohibited by law. It is essential to adhere to these guidelines to ensure compliance with legal and ethical standards in research and experimentation.