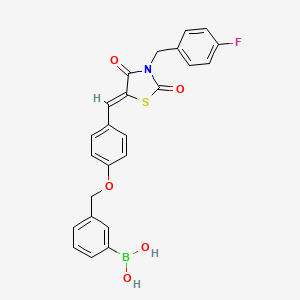
HA130
Overview
Description
HA130 ([3-[[4-[(Z)-[3-[(4-fluorophenyl)methyl]-2,4-dioxo-1,3-thiazolidin-5-ylidene]methyl]phenoxy]methyl]phenyl]boronic acid) is a boronic acid-containing thiazolidinedione derivative and a potent, selective inhibitor of autotaxin (ATX), an enzyme critical for lysophosphatidic acid (LPA) synthesis . This compound targets the ATX catalytic site Thr209, exhibiting an IC50 of 28 nM in the presence of lysophosphatidylcholine (LPC) . It suppresses LPA production, modulates inflammatory pathways, and demonstrates therapeutic efficacy across diverse disease models, including hepatic encephalopathy (HE), neuroinflammation, fibrosis, and viral infections .
In HE models, this compound reduces ammonia-induced neuroinflammation, restores blood-brain barrier integrity, and lowers liver enzymes (AST, ALT) . In neuroepithelial organoids, this compound alters morphogenesis by inhibiting LPA synthesis, mimicking retinoic acid (RA) effects and suppressing trans-/cis-fusion events . This compound also inhibits ATX-driven melanoma cell migration and fibrotic responses in cardiac fibroblasts .
Preparation Methods
HA130 is synthesized through a series of chemical reactions involving the modification of boronic acid groups. The synthetic route typically involves the interaction between boronic acid and threonine hydroxyl oxygen, inspired by the proteasome inhibitor bortezomib . The industrial production of this compound involves stringent quality control measures to ensure high purity and efficacy .
Chemical Reactions Analysis
Mechanism of Enzymatic Inhibition
HA130 exhibits mixed-type inhibition kinetics against ATX:
Parameter | Value/Effect | Source |
---|---|---|
V~max~ | Reduced by 45% | |
K~m~ | Increased 3-fold | |
Reversibility | Full activity restoration after washout |
This indicates this compound binds both the free enzyme and enzyme-substrate complex, altering substrate affinity and catalytic turnover .
Selectivity Profile
This compound shows high specificity for ATX over structurally similar enzymes:
Enzyme | Activity at 10 μM this compound | Significance |
---|---|---|
Proteasome | No inhibition | Excludes off-target effects |
NPP1 | No inhibition | Confirms ATX-specificity |
Alkaline Phosphatase | No effect | Validates mechanistic focus |
No cytotoxicity was observed in cell viability assays .
In Vivo Pharmacodynamic Effects
This compound rapidly modulates lysophosphatidic acid (LPA) levels in mice:
-
Plasma LPA reduction : 48% decrease within 2 minutes post-injection (1 nmol/g dose) .
-
Kinetics : Plasma this compound concentration peaks at 0.35 μM within 10 minutes, correlating with LPA decline (R² = 0.751) .
-
Dynamic balance : Sustained LPA levels depend on ATX-mediated synthesis and lipid phosphate phosphatase (LPP)-driven degradation .
Chemical Stability and Solubility
Property | Value |
---|---|
Solubility | 93 mg/mL in DMSO (200.73 mM) |
Aqueous | Insoluble |
Ethanol | Insoluble |
Therapeutic Implications
This compound’s ability to disrupt ATX-LPA signaling has been explored in:
-
Cancer microenvironments : Alters lipid homeostasis in pancreatic cancer-associated fibroblasts .
-
Uremic toxin clearance : Enhances β2-microglobulin and parathyroid hormone removal in hemodialysis .
This compound represents a paradigm for boronic acid-driven enzyme inhibition, combining reversible binding, high specificity, and rapid in vivo efficacy. Its chemical reactivity centers on the boronic acid group’s interaction with ATX’s catalytic threonine, making it a valuable tool for studying LPA-driven pathologies.
Scientific Research Applications
Hemoperfusion Therapy
Overview
HA130 is primarily utilized in hemoperfusion therapy to improve the quality of life for patients with chronic kidney disease (CKD) and end-stage renal disease (ESRD). The therapy effectively removes middle to large uremic toxins, such as beta-2 microglobulin (β2M) and parathyroid hormone (PTH), which are typically elevated in patients undergoing dialysis.
Mechanism of Action
The this compound disposable hemoperfusion cartridge is designed with a neutral macroporous resin that captures targeted molecules ranging from 5 kDa to 30 kDa through hydrophobic interactions and van der Waals forces. This technology allows for the efficient removal of protein-bound toxins from the blood during hemodialysis.
Clinical Benefits
- Improved Quality of Life: Patients receiving this compound therapy report significant relief from symptoms such as uremic pruritus and restless legs syndrome, leading to enhanced sleep quality .
- Extended Survival Rates: Studies indicate that integrating this compound with standard hemodialysis can extend patient life expectancy by approximately 2.87 years .
- Reduction in Complications: The therapy has been shown to decrease inflammation markers (hs-CRP, IL-6, TNF-α) and improve overall patient well-being .
Autotaxin Inhibition
Overview
this compound has been identified as a potent inhibitor of autotaxin, an enzyme involved in the production of lysophosphatidic acid (LPA), which plays a role in various pathological conditions including cancer and fibrosis.
Inhibition Mechanism
this compound acts as a mixed-type inhibitor with an IC50 value of approximately 28 nM, demonstrating a significant increase in potency compared to earlier compounds . The compound reduces both the maximum reaction rate (Vmax) and substrate affinity (Km), indicating its effectiveness in modulating autotaxin activity.
Research Findings
- Plasma LPA Levels: Administration of this compound leads to a rapid decrease in plasma LPA levels, confirming its role in regulating LPA turnover . In experimental models, this compound administration resulted in a 48% reduction in plasma LPA levels within minutes post-injection.
- Potential Therapeutic Applications: Given its inhibitory effects on autotaxin, this compound may have implications for treating conditions associated with high LPA levels, such as certain cancers and fibrotic diseases.
Case Studies
Mechanism of Action
HA130 exerts its effects by inhibiting the enzymatic activity of autotaxin, thereby reducing the production of lysophosphatidic acid. This inhibition affects various cellular processes, including cell proliferation, migration, and cytokine production . The molecular targets involved include the autotaxin enzyme and its associated receptors on T cells .
Comparison with Similar Compounds
Pharmacological and Structural Profiles
HA130 is benchmarked against other ATX inhibitors, including HA51, HA155, and the BoA series (Table 1).
Table 1: Comparative Pharmacological Profiles of this compound and Similar ATX Inhibitors
- HA51 : A carboxylic acid derivative with 38-fold lower potency than this compound due to weaker interactions with ATX’s catalytic pocket .
- HA155 : Structural optimization of this compound with para-boronic acid substitution enhances potency (IC50 = 5.7 nM) but retains poor metabolic stability .
- BoA Series: Boronic acid derivatives (e.g., 3BoA) inhibit ATX via non-catalytic site binding (Trp275), showing variable efficacy depending on substituent position .
Efficacy in Disease Models
This compound outperforms other compounds in multi-disease applications (Table 2).
Table 2: Efficacy of this compound and Comparator Compounds in Disease Models
- Neuroepithelial Organoids: this compound mimics RA by suppressing LPA synthesis, shifting organoid topology from multi-lobular "wiffle balls" to spherical structures. In contrast, Y-27632 (a ROCK inhibitor) reduces fusion rates but lacks direct ATX inhibition .
- Fibrosis : this compound downregulates fibronectin, COL1A1, and αSMA in cardiac fibroblasts, demonstrating broader anti-fibrotic effects than HA155 or BoA compounds .
- PRRSV Infection : this compound uniquely alleviates fever and weight loss in piglets, highlighting its anti-inflammatory versatility .
Limitations and Pharmacokinetic Challenges
- Metabolic Stability : this compound and HA155 exhibit short half-lives (<5 minutes in mice), limiting clinical translation despite high potency .
Biological Activity
HA130 is a potent and selective inhibitor of autotaxin, an enzyme that plays a crucial role in the production of lysophosphatidic acid (LPA), a lipid mediator involved in various physiological and pathological processes. This article delves into the biological activity of this compound, highlighting its mechanisms, effects in clinical studies, and potential therapeutic applications.
This compound functions as a reversible inhibitor of autotaxin with an IC50 value of 28 nM , effectively blocking the hydrolysis of substrates like bis-pNPP. It demonstrates selectivity by exhibiting no significant activity against other enzymes such as the proteasome or alkaline phosphatase . The inhibition of autotaxin leads to decreased levels of LPA, which is implicated in cell migration, inflammation, and tumor progression.
In Vitro Studies
In vitro assays have shown that this compound inhibits autotaxin-mediated cell migration. For instance, in A2058 melanoma cell assays, this compound effectively reduced cell migration induced by LPA, suggesting its potential application in cancer therapy . Additionally, studies indicate that this compound can rapidly decrease plasma LPA levels when administered intravenously in animal models .
Clinical Research Findings
Recent clinical studies have explored the efficacy of this compound in various medical contexts, particularly in hemodialysis and chronic kidney disease management.
Case Study: Hemoperfusion with this compound
A significant study published in Blood Purification assessed the effects of this compound hemoperfusion combined with hemodialysis (HD) on patients with end-stage kidney disease (ESKD). This four-year randomized controlled trial involved 438 patients across multiple centers. Key findings include:
- Reduction in Uremic Toxins : Patients receiving this compound treatment exhibited lower levels of beta-2 microglobulin (β2M) and parathyroid hormone (PTH), indicating effective removal of middle-molecular-weight toxins.
- Improvement in Symptoms : The study reported a significant reduction in uremic pruritus symptoms among patients treated with this compound compared to standard HD .
- Quality of Life Enhancements : After six months of treatment, patients' nutritional status and quality of life scores improved significantly compared to those on standard HD protocols .
Summary of Clinical Findings
Study Parameter | HD Group (Control) | HD + this compound Group | Statistical Significance |
---|---|---|---|
β2M Levels (mg/L) | 30.5 ± 4.2 | 20.1 ± 3.8 | p < 0.01 |
PTH Levels (pg/mL) | 300 ± 50 | 150 ± 30 | p < 0.01 |
VAS Score (Pruritus) | 8.47 ± 0.82 | 6.50 ± 0.82 | p < 0.001 |
Potential Therapeutic Applications
The inhibition of autotaxin by this compound presents several therapeutic opportunities:
- Cancer Treatment : By reducing LPA levels, this compound may help inhibit tumor growth and metastasis.
- Inflammatory Diseases : Given LPA's role in inflammation, this compound could be beneficial in treating conditions such as arthritis or inflammatory bowel disease.
- Neurological Disorders : Research indicates that targeting the autotaxin-LPA axis may protect against blood-brain barrier disruption and mitochondrial dysfunction following ischemic events .
Q & A
Basic Research Questions
Q. What is the molecular mechanism by which HA130 inhibits autotaxin (ATX), and how does this affect lysophosphatidic acid (LPA) metabolism?
this compound selectively binds to ATX, a key enzyme in LPA synthesis, with an IC50 of 28 nM . By blocking ATX's enzymatic activity, this compound reduces plasma LPA levels, as shown in murine models where intravenous administration caused a rapid, concentration-dependent decline in LPA (e.g., 50% reduction within 10 minutes post-injection) . Methodologically, researchers should validate ATX inhibition using in vitro assays (e.g., fluorescence-based enzymatic activity tests) and correlate results with in vivo LPA measurements via LC-MS/MS .
Q. What are the standard protocols for preparing and storing this compound in experimental settings?
this compound is typically dissolved in DMSO (5 mg/mL stock solution) due to poor aqueous solubility (<1 mg/mL in water/ethanol) . For in vivo studies, ensure fresh preparation to avoid degradation, and store aliquots at -80°C for long-term stability. Dose-response experiments should span 31–2000 nM for organoid studies and 0.3–10 μM for hematopoiesis models . Include vehicle controls (DMSO-only) to account for solvent effects.
Advanced Research Questions
Q. How can experimental design address discrepancies in this compound's efficacy across different biological models (e.g., hematopoiesis vs. neuroepithelial organoids)?
Contradictions arise from model-specific variables:
- Concentration : this compound reduces CD41+ hematopoietic progenitor cells at 10 μM but alters organoid topology at 2 μM .
- Administration route : Intravenous delivery ensures systemic LPA suppression , while localized in vitro application may require titration for tissue penetration.
- Temporal dynamics : Measure LPA levels at multiple timepoints (e.g., 2, 10, 60 minutes post-injection) to capture kinetic differences . Standardize protocols using published IC50 values (28 nM for ATX inhibition ) and validate with orthogonal assays (e.g., qPCR for ATX-regulated genes like Enpp2 ).
Q. What statistical and analytical methods are recommended to interpret this compound's dose-dependent effects on LPA and cellular phenotypes?
- Regression analysis : Use linear/logistic regression to model relationships between this compound concentration and outcomes (e.g., LPA reduction in Figure C, R² = 0.751 ).
- ANOVA with post-hoc tests : Compare treatment groups in multi-concentration experiments (e.g., CD41+ cell proportions across 0–10 μM this compound ).
- Live imaging and topology quantification : For organoid studies, track fusion events and lobule counts using time-lapse microscopy and computational morphometrics .
Q. How can this compound be integrated into combinatorial therapies to enhance mechanistic insights (e.g., with retinoic acid in organoid studies)?
Co-treatment with retinoic acid (RA) and this compound in neuroepithelial organoids revealed synergistic suppression of trans-/cis-fusion events, shifting morphogenesis toward spherical lobule formation . Methodologically:
- Dose matrix design : Test this compound (31–2000 nM) ± RA to identify additive/synergistic effects.
- RNA-seq validation : Confirm pathway modulation (e.g., Enpp2 downregulation ).
- Control for off-target effects : Use ATX-knockout models or rescue experiments with exogenous LPA.
Q. Data Interpretation and Reproducibility
Q. How should researchers resolve contradictions in this compound's impact on clinical vs. preclinical outcomes?
While this compound reduces LPA in mice within minutes , clinical translation for dialysis patients focuses on uremic toxin clearance (e.g., β2-microglobulin) via hemoperfusion, not direct ATX inhibition . To bridge this gap:
- Cross-validate biomarkers : Measure both LPA and surrogate endpoints (e.g., cytokine levels) in preclinical-clinical parallel studies.
- Adjust dosing regimens : Clinical this compound use (e.g., weekly hemoperfusion ) differs from acute preclinical models; consider chronic dosing studies in animals.
Q. What are the critical controls for ensuring this compound-specific effects in complex systems (e.g., co-cultures or in vivo microenvironments)?
- Pharmacological controls : Use alternative ATX inhibitors (e.g., PF-8380) to confirm target specificity.
- Genetic controls : Employ ATX-knockout models or siRNA-mediated ATX silencing.
- Solvent controls : Account for DMSO cytotoxicity, especially in long-term cultures (>24 hours).
Q. Methodological Tables
Table 1. Key this compound Parameters for Experimental Design
Parameter | Value/Range | Evidence Source |
---|---|---|
IC50 (ATX inhibition) | 28 nM | |
In vivo LPA half-life | <10 minutes (post-IV) | |
Solubility | 5 mg/mL in DMSO | |
Effective in vitro dose | 0.3–10 μM |
Table 2. Common Pitfalls and Solutions
Properties
IUPAC Name |
[4-[[4-[(Z)-[3-[(4-fluorophenyl)methyl]-2,4-dioxo-1,3-thiazolidin-5-ylidene]methyl]phenoxy]methyl]phenyl]boronic acid | |
---|---|---|
Source | PubChem | |
URL | https://pubchem.ncbi.nlm.nih.gov | |
Description | Data deposited in or computed by PubChem | |
InChI |
InChI=1S/C24H19BFNO5S/c26-20-9-3-17(4-10-20)14-27-23(28)22(33-24(27)29)13-16-5-11-21(12-6-16)32-15-18-1-7-19(8-2-18)25(30)31/h1-13,30-31H,14-15H2/b22-13- | |
Source | PubChem | |
URL | https://pubchem.ncbi.nlm.nih.gov | |
Description | Data deposited in or computed by PubChem | |
InChI Key |
BRWUZCBSWABPMR-XKZIYDEJSA-N | |
Source | PubChem | |
URL | https://pubchem.ncbi.nlm.nih.gov | |
Description | Data deposited in or computed by PubChem | |
Canonical SMILES |
B(C1=CC=C(C=C1)COC2=CC=C(C=C2)C=C3C(=O)N(C(=O)S3)CC4=CC=C(C=C4)F)(O)O | |
Source | PubChem | |
URL | https://pubchem.ncbi.nlm.nih.gov | |
Description | Data deposited in or computed by PubChem | |
Isomeric SMILES |
B(C1=CC=C(C=C1)COC2=CC=C(C=C2)/C=C\3/C(=O)N(C(=O)S3)CC4=CC=C(C=C4)F)(O)O | |
Source | PubChem | |
URL | https://pubchem.ncbi.nlm.nih.gov | |
Description | Data deposited in or computed by PubChem | |
Molecular Formula |
C24H19BFNO5S | |
Source | PubChem | |
URL | https://pubchem.ncbi.nlm.nih.gov | |
Description | Data deposited in or computed by PubChem | |
DSSTOX Substance ID |
DTXSID40676768 | |
Record name | [4-({4-[(Z)-{3-[(4-Fluorophenyl)methyl]-2,4-dioxo-1,3-thiazolidin-5-ylidene}methyl]phenoxy}methyl)phenyl]boronic acid | |
Source | EPA DSSTox | |
URL | https://comptox.epa.gov/dashboard/DTXSID40676768 | |
Description | DSSTox provides a high quality public chemistry resource for supporting improved predictive toxicology. | |
Molecular Weight |
463.3 g/mol | |
Source | PubChem | |
URL | https://pubchem.ncbi.nlm.nih.gov | |
Description | Data deposited in or computed by PubChem | |
CAS No. |
1229652-21-4 | |
Record name | [4-({4-[(Z)-{3-[(4-Fluorophenyl)methyl]-2,4-dioxo-1,3-thiazolidin-5-ylidene}methyl]phenoxy}methyl)phenyl]boronic acid | |
Source | EPA DSSTox | |
URL | https://comptox.epa.gov/dashboard/DTXSID40676768 | |
Description | DSSTox provides a high quality public chemistry resource for supporting improved predictive toxicology. | |
Retrosynthesis Analysis
AI-Powered Synthesis Planning: Our tool employs the Template_relevance Pistachio, Template_relevance Bkms_metabolic, Template_relevance Pistachio_ringbreaker, Template_relevance Reaxys, Template_relevance Reaxys_biocatalysis model, leveraging a vast database of chemical reactions to predict feasible synthetic routes.
One-Step Synthesis Focus: Specifically designed for one-step synthesis, it provides concise and direct routes for your target compounds, streamlining the synthesis process.
Accurate Predictions: Utilizing the extensive PISTACHIO, BKMS_METABOLIC, PISTACHIO_RINGBREAKER, REAXYS, REAXYS_BIOCATALYSIS database, our tool offers high-accuracy predictions, reflecting the latest in chemical research and data.
Strategy Settings
Precursor scoring | Relevance Heuristic |
---|---|
Min. plausibility | 0.01 |
Model | Template_relevance |
Template Set | Pistachio/Bkms_metabolic/Pistachio_ringbreaker/Reaxys/Reaxys_biocatalysis |
Top-N result to add to graph | 6 |
Feasible Synthetic Routes
Disclaimer and Information on In-Vitro Research Products
Please be aware that all articles and product information presented on BenchChem are intended solely for informational purposes. The products available for purchase on BenchChem are specifically designed for in-vitro studies, which are conducted outside of living organisms. In-vitro studies, derived from the Latin term "in glass," involve experiments performed in controlled laboratory settings using cells or tissues. It is important to note that these products are not categorized as medicines or drugs, and they have not received approval from the FDA for the prevention, treatment, or cure of any medical condition, ailment, or disease. We must emphasize that any form of bodily introduction of these products into humans or animals is strictly prohibited by law. It is essential to adhere to these guidelines to ensure compliance with legal and ethical standards in research and experimentation.