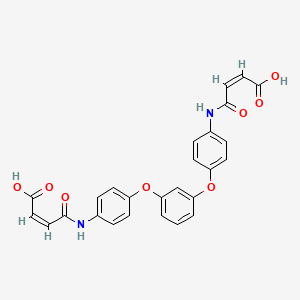
H2L5186303
Overview
Description
H2L5186303 is a potent and selective antagonist of the lysophosphatidic acid 2 receptor. This compound has shown significant potential in various scientific research fields due to its high specificity and efficacy. It is primarily used in studies related to lysophosphatidic acid signaling pathways, which are crucial in various physiological and pathological processes.
Mechanism of Action
H2L5186303, also known as “4,4’-[1,3-Phenylenebis(oxy-4,1-phenyleneimino)]bis[4-oxo-2-Butenoic acid” or “(Z)-4-[4-[3-[4-[[(Z)-3-Carboxyprop-2-enoyl]amino]phenoxy]phenoxy]anilino]-4-oxobut-2-enoic acid”, is a potent and selective antagonist of the Lysophosphatidic Acid 2 (LPA2) receptor .
Target of Action
The primary target of this compound is the Lysophosphatidic Acid 2 (LPA2) receptor . This receptor is part of the G protein-coupled receptor family and plays a crucial role in various biological processes, including cell proliferation, platelet aggregation, smooth muscle contraction, and chemotaxis .
Mode of Action
This compound acts as an antagonist at the LPA2 receptor, meaning it binds to the receptor and blocks its activation by LPA . The IC50 value, a measure of the effectiveness of the compound in inhibiting biological function, is 9 nM for the LPA2 receptor .
Biochemical Pathways
The LPA2 receptor is involved in several biochemical pathways. When LPA binds to the LPA2 receptor, it activates various downstream signaling pathways, including the phospholipase C (PLC), mitogen-activated protein kinase (MAPK), and phosphatidylinositol 3-kinase (PI3K) pathways . By blocking the LPA2 receptor, this compound can inhibit these pathways and their downstream effects .
Pharmacokinetics
The compound is soluble in dmso, which suggests it could be administered in a solution for in vivo studies .
Result of Action
This compound has been shown to have a suppressive effect on allergic responses when administered before antigen sensitization or challenge . It can suppress airway hyper-responsiveness, inflammatory cytokine levels, mucin production, and eosinophil numbers .
Action Environment
The action of this compound can be influenced by various environmental factors. For instance, the presence of allergens can affect the efficacy of the compound, as shown in a study where this compound was administered before antigen sensitization or challenge . The results of this study suggest that the timing of administration can significantly impact the compound’s efficacy .
Biochemical Analysis
Biochemical Properties
H2L5186303 plays a significant role in biochemical reactions due to its ability to antagonize the LPA2 receptor . This interaction with the LPA2 receptor can influence various biochemical processes, including signal transduction, cell proliferation, and survival .
Cellular Effects
The effects of this compound on cells are primarily mediated through its interaction with the LPA2 receptor . By antagonizing this receptor, this compound can influence cell function, including impacts on cell signaling pathways, gene expression, and cellular metabolism .
Molecular Mechanism
The molecular mechanism of action of this compound involves its binding to the LPA2 receptor, thereby inhibiting its activation . This can lead to changes in gene expression and cellular signaling pathways, contributing to its effects on cell function .
Metabolic Pathways
This compound is involved in the lysophosphatidic acid signaling pathway due to its interaction with the LPA2 receptor
Transport and Distribution
The transport and distribution of this compound within cells and tissues are likely influenced by its physicochemical properties and its interaction with the LPA2 receptor
Subcellular Localization
The subcellular localization of this compound and its effects on activity or function are likely influenced by its interaction with the LPA2 receptor
Preparation Methods
Synthetic Routes and Reaction Conditions
The synthesis of H2L5186303 involves multiple steps, starting with the preparation of intermediate compounds. The key steps include:
Formation of the core structure: This involves the reaction of specific aromatic compounds under controlled conditions to form the core structure of this compound.
Functionalization: The core structure is then functionalized through various chemical reactions, including nitration, reduction, and esterification, to introduce the necessary functional groups.
Purification: The final product is purified using techniques such as recrystallization and chromatography to achieve high purity.
Industrial Production Methods
Industrial production of this compound follows similar synthetic routes but on a larger scale. The process is optimized for efficiency and cost-effectiveness, often involving automated systems for precise control of reaction conditions. The use of high-throughput purification methods ensures the consistent quality of the compound.
Chemical Reactions Analysis
Types of Reactions
H2L5186303 undergoes various chemical reactions, including:
Oxidation: The compound can be oxidized under specific conditions to form oxidized derivatives.
Reduction: Reduction reactions can be used to modify certain functional groups within the molecule.
Substitution: this compound can undergo substitution reactions, where specific atoms or groups within the molecule are replaced by others.
Common Reagents and Conditions
Oxidation: Common oxidizing agents include potassium permanganate and hydrogen peroxide.
Reduction: Reducing agents such as sodium borohydride and lithium aluminum hydride are often used.
Substitution: Various halogenating agents and nucleophiles are employed in substitution reactions.
Major Products
The major products formed from these reactions depend on the specific conditions and reagents used. For example, oxidation may yield hydroxylated or carboxylated derivatives, while reduction can produce amine or alcohol derivatives.
Scientific Research Applications
H2L5186303 has a wide range of applications in scientific research:
Chemistry: It is used to study the lysophosphatidic acid signaling pathways and their role in various chemical processes.
Biology: The compound is employed in research on cell signaling, migration, and proliferation, particularly in cancer biology.
Medicine: this compound is investigated for its potential therapeutic applications in diseases such as cancer, fibrosis, and inflammatory conditions.
Industry: The compound is used in the development of new drugs and therapeutic agents targeting lysophosphatidic acid receptors.
Comparison with Similar Compounds
H2L5186303 is unique due to its high selectivity and potency for the lysophosphatidic acid 2 receptor. Similar compounds include:
Ki16425: Another lysophosphatidic acid receptor antagonist, but with less selectivity.
AM095: A selective lysophosphatidic acid receptor antagonist with different receptor subtype specificity.
BrP-LPA: A broad-spectrum lysophosphatidic acid receptor antagonist with varying efficacy across different receptor subtypes.
This compound stands out due to its high specificity for the lysophosphatidic acid 2 receptor, making it particularly useful in studies focused on this receptor subtype .
If you have any specific questions or need further details, feel free to ask!
Properties
IUPAC Name |
(Z)-4-[4-[3-[4-[[(Z)-3-carboxyprop-2-enoyl]amino]phenoxy]phenoxy]anilino]-4-oxobut-2-enoic acid | |
---|---|---|
Source | PubChem | |
URL | https://pubchem.ncbi.nlm.nih.gov | |
Description | Data deposited in or computed by PubChem | |
InChI |
InChI=1S/C26H20N2O8/c29-23(12-14-25(31)32)27-17-4-8-19(9-5-17)35-21-2-1-3-22(16-21)36-20-10-6-18(7-11-20)28-24(30)13-15-26(33)34/h1-16H,(H,27,29)(H,28,30)(H,31,32)(H,33,34)/b14-12-,15-13- | |
Source | PubChem | |
URL | https://pubchem.ncbi.nlm.nih.gov | |
Description | Data deposited in or computed by PubChem | |
InChI Key |
HZFPOTBCYPWQSH-DZDAAMPGSA-N | |
Source | PubChem | |
URL | https://pubchem.ncbi.nlm.nih.gov | |
Description | Data deposited in or computed by PubChem | |
Canonical SMILES |
C1=CC(=CC(=C1)OC2=CC=C(C=C2)NC(=O)C=CC(=O)O)OC3=CC=C(C=C3)NC(=O)C=CC(=O)O | |
Source | PubChem | |
URL | https://pubchem.ncbi.nlm.nih.gov | |
Description | Data deposited in or computed by PubChem | |
Isomeric SMILES |
C1=CC(=CC(=C1)OC2=CC=C(C=C2)NC(=O)/C=C\C(=O)O)OC3=CC=C(C=C3)NC(=O)/C=C\C(=O)O | |
Source | PubChem | |
URL | https://pubchem.ncbi.nlm.nih.gov | |
Description | Data deposited in or computed by PubChem | |
Molecular Formula |
C26H20N2O8 | |
Source | PubChem | |
URL | https://pubchem.ncbi.nlm.nih.gov | |
Description | Data deposited in or computed by PubChem | |
Molecular Weight |
488.4 g/mol | |
Source | PubChem | |
URL | https://pubchem.ncbi.nlm.nih.gov | |
Description | Data deposited in or computed by PubChem | |
Retrosynthesis Analysis
AI-Powered Synthesis Planning: Our tool employs the Template_relevance Pistachio, Template_relevance Bkms_metabolic, Template_relevance Pistachio_ringbreaker, Template_relevance Reaxys, Template_relevance Reaxys_biocatalysis model, leveraging a vast database of chemical reactions to predict feasible synthetic routes.
One-Step Synthesis Focus: Specifically designed for one-step synthesis, it provides concise and direct routes for your target compounds, streamlining the synthesis process.
Accurate Predictions: Utilizing the extensive PISTACHIO, BKMS_METABOLIC, PISTACHIO_RINGBREAKER, REAXYS, REAXYS_BIOCATALYSIS database, our tool offers high-accuracy predictions, reflecting the latest in chemical research and data.
Strategy Settings
Precursor scoring | Relevance Heuristic |
---|---|
Min. plausibility | 0.01 |
Model | Template_relevance |
Template Set | Pistachio/Bkms_metabolic/Pistachio_ringbreaker/Reaxys/Reaxys_biocatalysis |
Top-N result to add to graph | 6 |
Feasible Synthetic Routes
Disclaimer and Information on In-Vitro Research Products
Please be aware that all articles and product information presented on BenchChem are intended solely for informational purposes. The products available for purchase on BenchChem are specifically designed for in-vitro studies, which are conducted outside of living organisms. In-vitro studies, derived from the Latin term "in glass," involve experiments performed in controlled laboratory settings using cells or tissues. It is important to note that these products are not categorized as medicines or drugs, and they have not received approval from the FDA for the prevention, treatment, or cure of any medical condition, ailment, or disease. We must emphasize that any form of bodily introduction of these products into humans or animals is strictly prohibited by law. It is essential to adhere to these guidelines to ensure compliance with legal and ethical standards in research and experimentation.