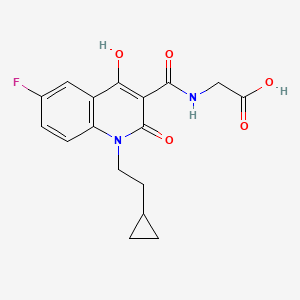
GSK360A
Overview
Description
GSK360A is a potent and orally active inhibitor of prolyl hydroxylase domain-containing enzymes. It specifically targets the hypoxia-inducible factor prolyl hydroxylase enzymes, which play a crucial role in the regulation of the hypoxia-inducible factor pathway. This compound has shown significant potential in protecting against various forms of ischemic injury, including myocardial infarction and stroke .
Preparation Methods
Synthetic Routes and Reaction Conditions
The synthesis of GSK360A involves multiple steps, starting from commercially available starting materialsThe reaction conditions typically involve the use of organic solvents, catalysts, and controlled temperatures to ensure high yield and purity .
Industrial Production Methods
Industrial production of this compound follows similar synthetic routes but on a larger scale. The process is optimized for efficiency and cost-effectiveness, often involving automated systems for precise control of reaction conditions. The final product undergoes rigorous quality control to meet pharmaceutical standards .
Chemical Reactions Analysis
Types of Reactions
GSK360A primarily undergoes substitution reactions due to the presence of reactive functional groups in its structure. It can also participate in oxidation and reduction reactions under specific conditions .
Common Reagents and Conditions
Common reagents used in the reactions involving this compound include organic solvents like dimethyl sulfoxide, catalysts such as palladium on carbon, and oxidizing agents like hydrogen peroxide. The reactions are typically carried out under controlled temperatures and inert atmospheres to prevent unwanted side reactions .
Major Products Formed
The major products formed from the reactions involving this compound depend on the specific reagents and conditions used. For example, oxidation reactions may yield hydroxylated derivatives, while substitution reactions can introduce various functional groups into the molecule .
Scientific Research Applications
GSK360A has a wide range of scientific research applications:
Mechanism of Action
GSK360A exerts its effects by inhibiting prolyl hydroxylase enzymes, which are responsible for the hydroxylation and subsequent degradation of hypoxia-inducible factor. By inhibiting these enzymes, this compound stabilizes hypoxia-inducible factor, leading to the activation of hypoxia-responsive genes. These genes play a crucial role in cellular adaptation to low oxygen conditions, promoting cell survival and tissue repair .
Comparison with Similar Compounds
Similar Compounds
DMOG (Dimethyloxalylglycine): Another prolyl hydroxylase inhibitor with similar effects on the hypoxia-inducible factor pathway.
Roxadustat: A clinically approved prolyl hydroxylase inhibitor used for the treatment of anemia in chronic kidney disease.
Uniqueness of GSK360A
This compound is unique due to its high potency and selectivity for prolyl hydroxylase enzymes. It has demonstrated significant efficacy in preclinical models of ischemic injury, making it a valuable tool for research and potential therapeutic applications .
Properties
IUPAC Name |
2-[[1-(2-cyclopropylethyl)-6-fluoro-4-hydroxy-2-oxoquinoline-3-carbonyl]amino]acetic acid | |
---|---|---|
Source | PubChem | |
URL | https://pubchem.ncbi.nlm.nih.gov | |
Description | Data deposited in or computed by PubChem | |
InChI |
InChI=1S/C17H17FN2O5/c18-10-3-4-12-11(7-10)15(23)14(16(24)19-8-13(21)22)17(25)20(12)6-5-9-1-2-9/h3-4,7,9,23H,1-2,5-6,8H2,(H,19,24)(H,21,22) | |
Source | PubChem | |
URL | https://pubchem.ncbi.nlm.nih.gov | |
Description | Data deposited in or computed by PubChem | |
InChI Key |
TYHRZQVUPPODPT-UHFFFAOYSA-N | |
Source | PubChem | |
URL | https://pubchem.ncbi.nlm.nih.gov | |
Description | Data deposited in or computed by PubChem | |
Canonical SMILES |
C1CC1CCN2C3=C(C=C(C=C3)F)C(=C(C2=O)C(=O)NCC(=O)O)O | |
Source | PubChem | |
URL | https://pubchem.ncbi.nlm.nih.gov | |
Description | Data deposited in or computed by PubChem | |
Molecular Formula |
C17H17FN2O5 | |
Source | PubChem | |
URL | https://pubchem.ncbi.nlm.nih.gov | |
Description | Data deposited in or computed by PubChem | |
DSSTOX Substance ID |
DTXSID10715754 | |
Record name | N-[1-(2-Cyclopropylethyl)-6-fluoro-4-hydroxy-2-oxo-1,2-dihydroquinoline-3-carbonyl]glycine | |
Source | EPA DSSTox | |
URL | https://comptox.epa.gov/dashboard/DTXSID10715754 | |
Description | DSSTox provides a high quality public chemistry resource for supporting improved predictive toxicology. | |
Molecular Weight |
348.32 g/mol | |
Source | PubChem | |
URL | https://pubchem.ncbi.nlm.nih.gov | |
Description | Data deposited in or computed by PubChem | |
CAS No. |
931399-19-8 | |
Record name | N-[1-(2-Cyclopropylethyl)-6-fluoro-4-hydroxy-2-oxo-1,2-dihydroquinoline-3-carbonyl]glycine | |
Source | EPA DSSTox | |
URL | https://comptox.epa.gov/dashboard/DTXSID10715754 | |
Description | DSSTox provides a high quality public chemistry resource for supporting improved predictive toxicology. | |
Retrosynthesis Analysis
AI-Powered Synthesis Planning: Our tool employs the Template_relevance Pistachio, Template_relevance Bkms_metabolic, Template_relevance Pistachio_ringbreaker, Template_relevance Reaxys, Template_relevance Reaxys_biocatalysis model, leveraging a vast database of chemical reactions to predict feasible synthetic routes.
One-Step Synthesis Focus: Specifically designed for one-step synthesis, it provides concise and direct routes for your target compounds, streamlining the synthesis process.
Accurate Predictions: Utilizing the extensive PISTACHIO, BKMS_METABOLIC, PISTACHIO_RINGBREAKER, REAXYS, REAXYS_BIOCATALYSIS database, our tool offers high-accuracy predictions, reflecting the latest in chemical research and data.
Strategy Settings
Precursor scoring | Relevance Heuristic |
---|---|
Min. plausibility | 0.01 |
Model | Template_relevance |
Template Set | Pistachio/Bkms_metabolic/Pistachio_ringbreaker/Reaxys/Reaxys_biocatalysis |
Top-N result to add to graph | 6 |
Feasible Synthetic Routes
Disclaimer and Information on In-Vitro Research Products
Please be aware that all articles and product information presented on BenchChem are intended solely for informational purposes. The products available for purchase on BenchChem are specifically designed for in-vitro studies, which are conducted outside of living organisms. In-vitro studies, derived from the Latin term "in glass," involve experiments performed in controlled laboratory settings using cells or tissues. It is important to note that these products are not categorized as medicines or drugs, and they have not received approval from the FDA for the prevention, treatment, or cure of any medical condition, ailment, or disease. We must emphasize that any form of bodily introduction of these products into humans or animals is strictly prohibited by law. It is essential to adhere to these guidelines to ensure compliance with legal and ethical standards in research and experimentation.