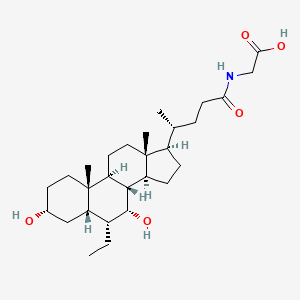
Glyco-obeticholic acid
Overview
Description
Glyco-obeticholic acid (Glyco-OCA) is the glycine-conjugated active metabolite of obeticholic acid (OCA), a semisynthetic bile acid derivative and potent farnesoid X receptor (FXR) agonist . OCA, initially developed for primary biliary cholangitis (PBC) and nonalcoholic steatohepatitis (NASH), undergoes hepatic conjugation with glycine to form Glyco-OCA, which retains FXR agonist activity . This metabolite is secreted into bile and participates in enterohepatic recirculation, where gut microbiota deconjugate it back to OCA for reabsorption or excretion . Glyco-OCA’s molecular formula is C₂₈H₄₇NO₅, with a molecular weight of 477.68 g/mol and CAS number 863239-60-5 . Its role in OCA’s therapeutic efficacy highlights the importance of metabolite activity in FXR-targeted therapies.
Preparation Methods
Synthetic Routes and Reaction Conditions: The synthesis of Glyco-Obeticholic Acid involves the conjugation of Obeticholic Acid with glycine. The process typically includes the activation of the carboxyl group of Obeticholic Acid, followed by its reaction with glycine under basic conditions. The reaction is usually carried out in an organic solvent such as dimethylformamide or dichloromethane .
Industrial Production Methods: Industrial production of this compound follows similar synthetic routes but on a larger scale. The process involves optimizing reaction conditions to maximize yield and purity. Techniques such as high-performance liquid chromatography are employed to purify the final product .
Chemical Reactions Analysis
Enterohepatic Recirculation
Glyco-OBET undergoes enterohepatic recirculation:
-
Biliary secretion : Conjugated G-OCA is secreted into bile .
-
Intestinal deconjugation : Gut microbiota hydrolyze G-OCA back to OCA in the ileum and colon .
-
Reabsorption : Free OCA is reabsorbed and reconjugated in the liver .
Key Enzymatic Processes
Physicochemical Stability
-
Thermal stability : Decomposition temperature undetermined, but stable under standard storage (2–8°C) .
-
Photostability : No data reported; assumed stable in opaque packaging .
-
Hydrolytic stability : Resistant to hydrolysis in plasma but susceptible to microbial degradation in the gut .
Analytical Stability
-
Plasma samples : Stable for 60 days at –70°C, 10 hours at room temperature, and 58 hours post-processing .
LC-MS/MS Quantification
A validated method for simultaneous detection of OCA and G-OCA in human plasma :
Parameter | OCA | G-OCA |
---|---|---|
Linearity range (ng/mL) | 0.50–100.00 | 0.50–100.00 |
Recovery (%) | 85.08 | 81.16 |
Matrix effect (%CV) | <5 | <5 |
LLOQ (ng/mL) | 0.50 | 0.50 |
Chromatographic conditions :
-
Column : Poroshell 120 EC-C18 (2.1 × 50 mm).
Pharmacokinetic Interactions
Dose-Dependent Accumulation
-
G-OCA exposure increases disproportionately at higher OCA doses .
-
Metabolite-to-parent ratio : 13.8 for G-OCA vs. 12.3 for tauro-OBET .
Toxicological Considerations
Scientific Research Applications
Treatment of Primary Biliary Cholangitis (PBC)
Glyco-obeticholic acid is primarily indicated for the treatment of primary biliary cholangitis, especially in patients who do not respond adequately to ursodeoxycholic acid. Clinical trials have demonstrated that obeticholic acid significantly reduces alkaline phosphatase (ALP) levels, a key marker in PBC management.
- Clinical Efficacy : In a phase III trial involving 216 patients, 59% of those treated with obeticholic acid achieved a significant reduction in ALP levels compared to placebo . The mean reduction in ALP was reported to be 246 U/L in treated patients versus an increase in the placebo group .
Study | Treatment Group | Responders (%) | Mean ALP Reduction (U/L) |
---|---|---|---|
Phase III | Obeticholic Acid | 59 | 246 |
Placebo | 7 | -17 |
Non-Alcoholic Steatohepatitis (NASH)
This compound is under investigation for its potential benefits in treating non-alcoholic steatohepatitis. Initial studies suggest that it may help reduce liver fibrosis and improve hepatic outcomes.
- Case Study Findings : A recent real-world study indicated a 63% reduction in hospitalization rates for hepatic decompensation among patients treated with obeticholic acid compared to those who were not . This highlights its potential role in improving long-term liver health.
Safety and Tolerability
While this compound has shown promising results, safety remains a critical concern. Common side effects include pruritus and potential liver failure in advanced cirrhosis cases.
Mechanism of Action
Glyco-Obeticholic Acid exerts its effects primarily through the activation of the farnesoid X receptor. This receptor is a nuclear receptor that regulates bile acid synthesis, lipid metabolism, and glucose homeostasis. Activation of the farnesoid X receptor by this compound leads to the suppression of bile acid synthesis, reduction of hepatic inflammation, and attenuation of fibrosis .
Comparison with Similar Compounds
Structural and Pharmacokinetic Differences
Glyco-OCA is compared below with OCA, tauro-obeticholic acid (Tauro-OCA), ursodeoxycholic acid (UDCA), and other FXR agonists.
*Tauro-OCA’s molecular weight inferred from OCA (C₂₆H₄₄O₄) + taurine (C₂H₇NO₃S).
Key Observations :
- Conjugation Type: Glyco-OCA and Tauro-OCA differ in amino acid conjugation (glycine vs. taurine), influencing solubility and enterohepatic recycling. Both metabolites exhibit overlapping pharmacokinetic (PK) profiles with OCA but may vary in absorption efficiency .
- Bioequivalence : A study comparing OCA, Glyco-OCA, and Tauro-OCA under fed/fasting conditions demonstrated bioequivalence between test and reference formulations, confirming similar systemic exposure .
- Enterohepatic Recirculation : Glyco-OCA and Tauro-OCA are secreted into bile, reabsorbed in the intestine, and contribute to prolonged FXR activation, enhancing OCA’s therapeutic effect .
Pharmacological Activity and Clinical Efficacy
Clinical Insights :
Biological Activity
Glyco-obeticholic acid (glyco-OCA) is an active metabolite of obeticholic acid (OCA), a semisynthetic bile acid that acts as a potent and selective agonist of the farnesoid X receptor (FXR). This article explores the biological activity of glyco-OCA, focusing on its pharmacokinetics, therapeutic effects, and safety profile based on diverse research findings.
Overview of this compound
Glyco-OCA is formed through the conjugation of OCA with glycine, which enhances its solubility and bioavailability. As an FXR agonist, glyco-OCA plays a crucial role in regulating bile acid homeostasis, lipid metabolism, and glucose metabolism. Understanding its biological activity is essential for evaluating its therapeutic potential in liver diseases.
Absorption and Metabolism
The pharmacokinetic properties of OCA and its metabolites, including glyco-OCA, have been extensively studied. A notable study involving 160 healthy volunteers reported the concentration-time profiles for OCA and its metabolites after administration. The results indicated that glyco-OCA concentrations were significant following multiple doses of OCA, suggesting that it is an important metabolite in humans .
Parameter | Glyco-OCA | OCA |
---|---|---|
EC50 (FXR Activation) | Similar to OCA | ~100-fold lower than CDCA |
Primary Metabolite | Yes | No |
Major Conjugation | Glycine | Taurine |
Efficacy in Liver Diseases
Glyco-OCA has shown promise in treating chronic liver diseases, particularly primary biliary cholangitis (PBC) and nonalcoholic steatohepatitis (NASH). In clinical trials, OCA demonstrated significant reductions in alkaline phosphatase (ALP) levels among patients with PBC who had inadequate responses to ursodeoxycholic acid therapy. The trials indicated that glyco-OCA contributes to the overall efficacy of OCA by enhancing its therapeutic effects .
- Clinical Trial Findings :
- Study Design : Double-blind trial with 165 patients.
- Dosage : Patients received 10 mg, 25 mg, or 50 mg doses of OCA.
- Results :
Case Studies
-
Case Study on NASH :
- A recent study highlighted that patients with NASH exhibited improved liver histology when treated with OCA. The upregulation of alternative bile acid synthesis pathways was identified as a key factor influencing treatment response, suggesting that glyco-OCA may enhance therapeutic outcomes through metabolic modulation .
- Long-term Efficacy :
Safety Profile
The safety profile of glyco-OCA has been evaluated alongside that of OCA. Common adverse effects include pruritus, which was dose-dependent in clinical studies. The incidence rates were significantly lower in patients receiving the lowest dose (10 mg) compared to higher doses . Importantly, preclinical studies indicated that glyco-OCA and its parent compound did not exhibit genotoxicity or carcinogenicity in various assays .
Q & A
Basic Research Questions
Q. What is the structural and functional relationship between Glyco-obeticholic acid (G-OCA) and its parent compound, Obeticholic Acid (OCA)?
G-OCA is a glycine-conjugated metabolite of OCA, formed via phase II metabolism. Unlike OCA, G-OCA retains potent farnesoid X receptor (FXR) agonism while exhibiting distinct pharmacokinetic (PK) properties due to enhanced solubility and enterohepatic recirculation . This conjugation alters its absorption and distribution, making it a key contributor to OCA’s sustained pharmacological effects in cholestatic liver diseases .
Q. How does G-OCA activate FXR, and what downstream pathways are clinically relevant?
G-OCA binds to FXR, a nuclear receptor regulating bile acid homeostasis. Activation suppresses CYP7A1 (reducing bile acid synthesis) and upregulates BSEP (enhancing biliary excretion). These mechanisms reduce toxic bile acid accumulation, as demonstrated in phase III trials where G-OCA lowered alkaline phosphatase (ALP) by 21–25% in primary biliary cholangitis (PBC) patients . Target engagement is confirmed via reductions in γ-glutamyl transpeptidase (GGT) and immunoglobulin M (IgM) .
Q. What pharmacokinetic (PK) parameters differentiate G-OCA from OCA in clinical studies?
G-OCA exhibits dose-proportional systemic exposure with nonlinear accumulation at higher doses (e.g., 25 mg OCA increases G-OCA AUC0-24h 7.8-fold vs. baseline). Its enterohepatic recirculation prolongs half-life (t1/2 ≈ 12–24 hrs), contrasting with OCA’s shorter t1/2 (~1.5 hrs). Biliary secretion and fecal excretion dominate elimination .
Q. What validated analytical methods quantify G-OCA in biological matrices?
Liquid chromatography-tandem mass spectrometry (LC-MS/MS) with deuterated internal standards (e.g., this compound-d5) is the gold standard. This method achieves high sensitivity (LOQ ≤1 ng/mL) and specificity, critical for assessing enterohepatic recirculation and metabolite ratios in PK studies .
Advanced Research Questions
Q. How should clinical trials evaluate G-OCA’s efficacy in PBC while managing dose-limiting pruritus?
Trials should use adaptive designs with dose titration (e.g., starting at 5 mg, escalating to 10 mg) to balance efficacy (ALP reduction ≥15%) and tolerability. Pruritus incidence (56–68% in OCA trials ) requires preemptive stratification by itch severity scales (e.g., Visual Analog Scale) and rescue medications (e.g., bile acid sequestrants). Composite endpoints (ALP + bilirubin normalization) improve prognostic validity .
Q. What experimental models best elucidate tissue-specific FXR activation by G-OCA?
In vitro: Primary human hepatocytes and intestinal organoids assess gene regulation (e.g., SHP, BSEP). In vivo: Bile duct-ligated (BDL) rats and Abcb11<sup>-/-</sup> mice model cholestasis, showing G-OCA’s anti-fibrotic effects via reduced collagen deposition . Co-administration with ursodeoxycholic acid (UDCA) mimics clinical regimens .
Q. How do genetic polymorphisms in bile acid transporters influence G-OCA’s pharmacodynamics?
Variants in ABCB11 (BSEP) and SLCO1B1 (OATP1B1) alter hepatic uptake and efflux. Genotype-guided dosing may mitigate interpatient variability. For example, SLCO1B1 c.521T>C carriers show 30% lower G-OCA exposure, necessitating dose adjustments .
Q. What statistical approaches address contradictions between biochemical efficacy and adverse events in G-OCA trials?
Bayesian hierarchical models adjust for pruritus-related dropout rates, while sensitivity analyses (e.g., tipping-point analysis) quantify robustness of ALP improvements. In the POISE trial, 47% of OCA-treated patients achieved primary endpoints despite 16% discontinuation due to pruritus .
Q. How does enterohepatic recirculation complicate PK modeling of G-OCA?
Mechanistic models incorporate recycling loops (e.g., GI transit time, biliary secretion rates) and nonlinear clearance. Population PK analyses reveal covariates (e.g., body mass index, UDCA co-therapy) explaining 25% of variability in G-OCA exposure .
Q. What preclinical evidence supports G-OCA’s anti-fibrotic effects in non-cholestatic liver diseases?
In NASH models, G-OCA reduces hepatic collagen content by 40% via FXR-mediated suppression of TGF-β1 and TIMP-1. Synergy with PPARα agonists (e.g., fenofibrate) enhances steatosis resolution, suggesting combinatorial therapeutic potential .
Properties
IUPAC Name |
2-[[(4R)-4-[(3R,5S,6R,7R,8S,9S,10S,13R,14S,17R)-6-ethyl-3,7-dihydroxy-10,13-dimethyl-2,3,4,5,6,7,8,9,11,12,14,15,16,17-tetradecahydro-1H-cyclopenta[a]phenanthren-17-yl]pentanoyl]amino]acetic acid | |
---|---|---|
Source | PubChem | |
URL | https://pubchem.ncbi.nlm.nih.gov | |
Description | Data deposited in or computed by PubChem | |
InChI |
InChI=1S/C28H47NO5/c1-5-18-22-14-17(30)10-12-28(22,4)21-11-13-27(3)19(7-8-20(27)25(21)26(18)34)16(2)6-9-23(31)29-15-24(32)33/h16-22,25-26,30,34H,5-15H2,1-4H3,(H,29,31)(H,32,33)/t16-,17-,18-,19-,20+,21+,22+,25+,26-,27-,28-/m1/s1 | |
Source | PubChem | |
URL | https://pubchem.ncbi.nlm.nih.gov | |
Description | Data deposited in or computed by PubChem | |
InChI Key |
MTLPUOZJBFHNSO-FCWTVGIUSA-N | |
Source | PubChem | |
URL | https://pubchem.ncbi.nlm.nih.gov | |
Description | Data deposited in or computed by PubChem | |
Canonical SMILES |
CCC1C2CC(CCC2(C3CCC4(C(C3C1O)CCC4C(C)CCC(=O)NCC(=O)O)C)C)O | |
Source | PubChem | |
URL | https://pubchem.ncbi.nlm.nih.gov | |
Description | Data deposited in or computed by PubChem | |
Isomeric SMILES |
CC[C@@H]1[C@@H]2C[C@@H](CC[C@@]2([C@H]3CC[C@]4([C@H]([C@@H]3[C@@H]1O)CC[C@@H]4[C@H](C)CCC(=O)NCC(=O)O)C)C)O | |
Source | PubChem | |
URL | https://pubchem.ncbi.nlm.nih.gov | |
Description | Data deposited in or computed by PubChem | |
Molecular Formula |
C28H47NO5 | |
Source | PubChem | |
URL | https://pubchem.ncbi.nlm.nih.gov | |
Description | Data deposited in or computed by PubChem | |
Molecular Weight |
477.7 g/mol | |
Source | PubChem | |
URL | https://pubchem.ncbi.nlm.nih.gov | |
Description | Data deposited in or computed by PubChem | |
CAS No. |
863239-60-5 | |
Record name | Glyco-obeticholic acid | |
Source | ChemIDplus | |
URL | https://pubchem.ncbi.nlm.nih.gov/substance/?source=chemidplus&sourceid=0863239605 | |
Description | ChemIDplus is a free, web search system that provides access to the structure and nomenclature authority files used for the identification of chemical substances cited in National Library of Medicine (NLM) databases, including the TOXNET system. | |
Record name | GLYCO-OBETICHOLIC ACID | |
Source | FDA Global Substance Registration System (GSRS) | |
URL | https://gsrs.ncats.nih.gov/ginas/app/beta/substances/O2MZK6V9LQ | |
Description | The FDA Global Substance Registration System (GSRS) enables the efficient and accurate exchange of information on what substances are in regulated products. Instead of relying on names, which vary across regulatory domains, countries, and regions, the GSRS knowledge base makes it possible for substances to be defined by standardized, scientific descriptions. | |
Explanation | Unless otherwise noted, the contents of the FDA website (www.fda.gov), both text and graphics, are not copyrighted. They are in the public domain and may be republished, reprinted and otherwise used freely by anyone without the need to obtain permission from FDA. Credit to the U.S. Food and Drug Administration as the source is appreciated but not required. | |
Disclaimer and Information on In-Vitro Research Products
Please be aware that all articles and product information presented on BenchChem are intended solely for informational purposes. The products available for purchase on BenchChem are specifically designed for in-vitro studies, which are conducted outside of living organisms. In-vitro studies, derived from the Latin term "in glass," involve experiments performed in controlled laboratory settings using cells or tissues. It is important to note that these products are not categorized as medicines or drugs, and they have not received approval from the FDA for the prevention, treatment, or cure of any medical condition, ailment, or disease. We must emphasize that any form of bodily introduction of these products into humans or animals is strictly prohibited by law. It is essential to adhere to these guidelines to ensure compliance with legal and ethical standards in research and experimentation.