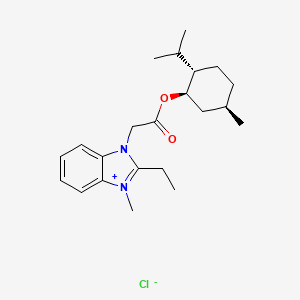
Gboxin
Overview
Description
Gboxin is a novel small molecule that specifically inhibits the growth of primary mouse and human glioblastoma cells. It is an oxidative phosphorylation inhibitor that targets the mitochondrial oxidative phosphorylation complexes, particularly the F0F1 ATP synthase complex . This compound has shown promising results in inhibiting glioblastoma growth without affecting normal cells, making it a potential therapeutic agent for glioblastoma treatment .
Mechanism of Action
Target of Action
Gboxin, an oxidative phosphorylation (OXPHOS) inhibitor, specifically targets the F0F1 ATPase complex V . This complex is a part of the mitochondrial oxidative phosphorylation complexes .
Mode of Action
This compound relies on its positive charge to associate with mitochondrial oxidative phosphorylation complexes . This association is dependent on the proton gradient of the inner mitochondrial membrane . This compound inhibits the activity of F0F1 ATP synthase , which is a crucial enzyme in the process of ATP synthesis.
Biochemical Pathways
The primary biochemical pathway affected by this compound is the oxidative phosphorylation (OXPHOS) pathway . By inhibiting the F0F1 ATP synthase, this compound disrupts the process of ATP synthesis, which is a key energy production mechanism in cells . This disruption compromises oxygen consumption in glioblastoma cells .
Pharmacokinetics
These factors lead to insufficient this compound accumulation at glioblastoma sites, limiting its clinical application . A biomimetic nanomedicine (hm-nps@g) has been developed by coating a cancer cell-mitochondria hybrid membrane (hm) on the surface of this compound-loaded nanoparticles . This approach improves the pharmacokinetic profile of this compound, enhancing its blood circulation and tumor accumulation .
Result of Action
This compound specifically inhibits the growth of primary mouse and human glioblastoma cells . It rapidly and irreversibly compromises oxygen consumption in glioblastoma cells . This leads to the eventual death of glioblastoma tumor cells .
Action Environment
The action of this compound is influenced by the unique physicochemical environment within glioblastoma stem cell (GSC) mitochondria . Normal body cells can evade the inhibitory effect of this compound on OXPHOS by opening the mitochondrial permeability transition pore (mPTP), which maintains a lower mitochondrial inner membrane potential . This mechanism prevents the significant impact of this compound on normal body cells .
Biochemical Analysis
Biochemical Properties
Gboxin is known to play a significant role in biochemical reactions, particularly in inhibiting the activity of the F0F1 ATPase complex V . This complex is a crucial component of the mitochondrial oxidative phosphorylation system, which is responsible for ATP synthesis .
Cellular Effects
This compound has been observed to have specific effects on various types of cells, particularly glioblastoma cells . It specifically inhibits the growth of these cells but does not affect the growth of mouse embryonic fibroblasts or neonatal astrocytes . This compound rapidly and irreversibly compromises oxygen consumption in glioblastoma cells .
Molecular Mechanism
The molecular mechanism of this compound involves its positive charge, which allows it to associate with mitochondrial oxidative phosphorylation complexes . This association is dependent on the proton gradient of the inner mitochondrial membrane . This compound inhibits the activity of the F0F1 ATP synthase, thereby disrupting oxidative phosphorylation .
Temporal Effects in Laboratory Settings
In laboratory settings, this compound has been observed to have a rapid and irreversible effect on glioblastoma cells . It quickly compromises oxygen consumption in these cells
Dosage Effects in Animal Models
It has been noted that this compound exhibits toxicity to established human cancer cell lines of diverse organ origin .
Metabolic Pathways
This compound is involved in the oxidative phosphorylation pathway, where it inhibits the F0F1 ATPase complex V . This complex is a key player in the synthesis of ATP, the main energy currency of cells .
Transport and Distribution
It is known that this compound relies on its positive charge to associate with mitochondrial oxidative phosphorylation complexes .
Subcellular Localization
This compound is known to accumulate inside the mitochondria of glioblastoma cells . This localization is crucial for its ability to inhibit the F0F1 ATPase complex V and disrupt oxidative phosphorylation .
Preparation Methods
The preparation of Gboxin involves several synthetic steps. The process begins with the reaction of o-phenylenediamine with N-propionaldehyde to prepare 2-ethyl-1H-benzimidazole. This intermediate undergoes N-alkylation with chloroacetic acid-L-menthyl ester to generate 2-(2-ethyl-1H-benzimidazole) acetic acid-L-menthyl ester. The next step involves methylation using methyl iodide to produce benzimidazolium salt. Finally, iodide ions are exchanged with chloride ions using an ion exchange resin to obtain the final product, this compound . This method is advantageous due to its short process route, mild conditions, simple operation, low equipment requirements, high purity, and high yield, making it suitable for industrial production .
Chemical Reactions Analysis
Gboxin undergoes various chemical reactions, including oxidation, reduction, and substitution. The compound’s positive charge allows it to associate with mitochondrial oxidative phosphorylation complexes in a manner dependent on the proton gradient of the inner mitochondrial membrane . Common reagents used in these reactions include o-phenylenediamine, N-propionaldehyde, chloroacetic acid-L-menthyl ester, and methyl iodide . The major products formed from these reactions are intermediates that lead to the final product, this compound .
Scientific Research Applications
Gboxin has several scientific research applications, particularly in the fields of chemistry, biology, medicine, and industry. In medicine, this compound is being studied for its potential to treat glioblastoma, a highly aggressive brain tumor. It specifically inhibits glioblastoma cell growth by targeting the mitochondrial oxidative phosphorylation complexes . In biology, this compound is used to study the metabolic needs of cancer cells and their reliance on oxidative phosphorylation for survival . In chemistry, this compound serves as a model compound for studying the synthesis and reactivity of benzimidazole derivatives . Additionally, this compound’s unique properties make it a valuable tool in the development of new therapeutic agents for cancer treatment .
Comparison with Similar Compounds
Gboxin is unique compared to other similar compounds due to its specific targeting of glioblastoma cells and its reliance on the proton gradient of the inner mitochondrial membrane for its activity . Similar compounds include other oxidative phosphorylation inhibitors, such as oligomycin and rotenone, which also target mitochondrial complexes but may not have the same specificity for glioblastoma cells . This compound’s ability to selectively inhibit glioblastoma cell growth without affecting normal cells sets it apart from these other compounds .
Properties
IUPAC Name |
[(1R,2S,5R)-5-methyl-2-propan-2-ylcyclohexyl] 2-(2-ethyl-3-methylbenzimidazol-3-ium-1-yl)acetate;chloride | |
---|---|---|
Source | PubChem | |
URL | https://pubchem.ncbi.nlm.nih.gov | |
Description | Data deposited in or computed by PubChem | |
InChI |
InChI=1S/C22H33N2O2.ClH/c1-6-21-23(5)18-9-7-8-10-19(18)24(21)14-22(25)26-20-13-16(4)11-12-17(20)15(2)3;/h7-10,15-17,20H,6,11-14H2,1-5H3;1H/q+1;/p-1/t16-,17+,20-;/m1./s1 | |
Source | PubChem | |
URL | https://pubchem.ncbi.nlm.nih.gov | |
Description | Data deposited in or computed by PubChem | |
InChI Key |
UBWVTCCKVGOTBG-VYZBTARASA-M | |
Source | PubChem | |
URL | https://pubchem.ncbi.nlm.nih.gov | |
Description | Data deposited in or computed by PubChem | |
Canonical SMILES |
CCC1=[N+](C2=CC=CC=C2N1CC(=O)OC3CC(CCC3C(C)C)C)C.[Cl-] | |
Source | PubChem | |
URL | https://pubchem.ncbi.nlm.nih.gov | |
Description | Data deposited in or computed by PubChem | |
Isomeric SMILES |
CCC1=[N+](C2=CC=CC=C2N1CC(=O)O[C@@H]3C[C@@H](CC[C@H]3C(C)C)C)C.[Cl-] | |
Source | PubChem | |
URL | https://pubchem.ncbi.nlm.nih.gov | |
Description | Data deposited in or computed by PubChem | |
Molecular Formula |
C22H33ClN2O2 | |
Source | PubChem | |
URL | https://pubchem.ncbi.nlm.nih.gov | |
Description | Data deposited in or computed by PubChem | |
Molecular Weight |
393.0 g/mol | |
Source | PubChem | |
URL | https://pubchem.ncbi.nlm.nih.gov | |
Description | Data deposited in or computed by PubChem | |
CAS No. |
2101315-36-8 | |
Record name | 1H-Benzimidazolium, 2-ethyl-1-methyl-3-[2-[[(1R,2S,5R)-5-methyl-2-(1-methylethyl)cyclohexyl]oxy]-2-oxoethyl]-, chloride (1:1) | |
Source | CAS Common Chemistry | |
URL | https://commonchemistry.cas.org/detail?cas_rn=2101315-36-8 | |
Description | CAS Common Chemistry is an open community resource for accessing chemical information. Nearly 500,000 chemical substances from CAS REGISTRY cover areas of community interest, including common and frequently regulated chemicals, and those relevant to high school and undergraduate chemistry classes. This chemical information, curated by our expert scientists, is provided in alignment with our mission as a division of the American Chemical Society. | |
Explanation | The data from CAS Common Chemistry is provided under a CC-BY-NC 4.0 license, unless otherwise stated. | |
Disclaimer and Information on In-Vitro Research Products
Please be aware that all articles and product information presented on BenchChem are intended solely for informational purposes. The products available for purchase on BenchChem are specifically designed for in-vitro studies, which are conducted outside of living organisms. In-vitro studies, derived from the Latin term "in glass," involve experiments performed in controlled laboratory settings using cells or tissues. It is important to note that these products are not categorized as medicines or drugs, and they have not received approval from the FDA for the prevention, treatment, or cure of any medical condition, ailment, or disease. We must emphasize that any form of bodily introduction of these products into humans or animals is strictly prohibited by law. It is essential to adhere to these guidelines to ensure compliance with legal and ethical standards in research and experimentation.