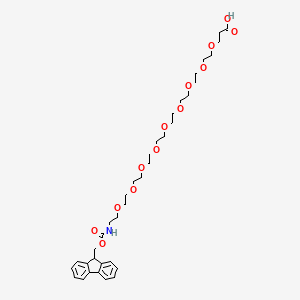
Fmoc-N-amido-PEG9-acid
Overview
Description
Fmoc-N-amido-PEG9-acid: is a polyethylene glycol (PEG) derivative that contains a fluorenylmethyloxycarbonyl (Fmoc)-protected amine and a terminal carboxylic acid. The hydrophilic PEG spacer increases solubility in aqueous media. The Fmoc group can be deprotected under basic conditions to obtain the free amine, which can be used for further conjugations. The terminal carboxylic acid can react with primary amine groups in the presence of activators to form a stable amide bond .
Scientific Research Applications
Chemistry:
Peptide Synthesis: Fmoc-N-amido-PEG9-acid is used as a linker in solid-phase peptide synthesis (SPPS) to introduce hydrophilic spacers between peptide chains.
Biology:
Protein Conjugation: It is used to conjugate proteins and peptides, enhancing their solubility and stability
Medicine:
Drug Delivery: The compound is employed in the development of antibody-drug conjugates (ADCs) for targeted drug delivery.
Industry:
Mechanism of Action
Target of Action
Fmoc-N-amido-PEG9-acid, also known as Fmoc-NH-PEG9-CH2CH2COOH, is primarily used as a linker in the synthesis of antibody-drug conjugates (ADCs) . The primary targets of this compound are therefore the antibodies to which it is conjugated. These antibodies are designed to bind specifically to antigens on the surface of target cells, such as cancer cells .
Mode of Action
The compound contains an Fmoc-protected amine and a terminal carboxylic acid . The Fmoc group can be deprotected under basic conditions to obtain the free amine, which can be used for further conjugations . The terminal carboxylic acid can react with primary amine groups in the presence of activators (e.g., EDC, or HATU) to form a stable amide bond . This allows the compound to be linked to antibodies, forming an ADC .
Biochemical Pathways
Instead, it serves to connect the antibody with a drug molecule in ADCs . The specific pathways affected would therefore depend on the drug molecule attached to the linker.
Pharmacokinetics
The pharmacokinetics of this compound would largely depend on the properties of the ADC of which it is a part . As a PEG linker, it increases the solubility of the ADC in aqueous media , which could enhance the bioavailability of the drug.
Result of Action
The result of the action of this compound is the formation of a stable ADC . The ADC can then bind to its target antigen on the cell surface, leading to internalization and release of the drug molecule within the cell . The specific molecular and cellular effects would depend on the mechanism of action of the drug molecule attached to the linker.
Action Environment
The action of this compound, as part of an ADC, can be influenced by various environmental factors. For example, the pH of the environment can affect the deprotection of the Fmoc group . Furthermore, the stability and efficacy of the ADC can be influenced by factors such as temperature and the presence of proteases.
Future Directions
Preparation Methods
Synthetic Routes and Reaction Conditions: The synthesis of Fmoc-N-amido-PEG9-acid typically involves the following steps:
Fmoc Protection: The amine group is protected using the Fmoc group.
PEGylation: The protected amine is then reacted with a PEG derivative to introduce the PEG spacer. .
Industrial Production Methods: Industrial production of this compound follows similar synthetic routes but on a larger scale. The process involves:
Bulk Synthesis: Large-scale synthesis of the Fmoc-protected amine and PEG derivatives.
Purification: The product is purified using techniques such as column chromatography or recrystallization to achieve high purity.
Quality Control: The final product undergoes rigorous quality control to ensure consistency and purity, often verified by NMR and HPLC analysis
Chemical Reactions Analysis
Types of Reactions:
Deprotection: The Fmoc group can be removed under basic conditions, typically using piperidine, to yield the free amine.
Amide Bond Formation: The terminal carboxylic acid can react with primary amines in the presence of coupling agents like EDC or HATU to form stable amide bonds
Common Reagents and Conditions:
Deprotection: Piperidine in DMF (dimethylformamide) is commonly used for Fmoc deprotection.
Coupling Agents: EDC, HATU, or DCC (dicyclohexylcarbodiimide) are used to activate the carboxylic acid for amide bond formation
Major Products:
Free Amine: Obtained after Fmoc deprotection.
Amide Conjugates: Formed by reacting the terminal carboxylic acid with primary amines
Comparison with Similar Compounds
Fmoc-N-amido-dPEG2-acid: A shorter PEG spacer with similar functional groups.
Fmoc-N-amido-dPEG24-acid: A longer PEG spacer with similar functional groups.
Uniqueness:
Hydrophilicity: The PEG9 spacer in Fmoc-N-amido-PEG9-acid provides an optimal balance of hydrophilicity and flexibility, making it suitable for a wide range of applications
Versatility: The compound’s ability to form stable amide bonds and its compatibility with various coupling agents make it highly versatile for bioconjugation and peptide synthesis
Properties
IUPAC Name |
3-[2-[2-[2-[2-[2-[2-[2-[2-[2-(9H-fluoren-9-ylmethoxycarbonylamino)ethoxy]ethoxy]ethoxy]ethoxy]ethoxy]ethoxy]ethoxy]ethoxy]ethoxy]propanoic acid | |
---|---|---|
Source | PubChem | |
URL | https://pubchem.ncbi.nlm.nih.gov | |
Description | Data deposited in or computed by PubChem | |
InChI |
InChI=1S/C36H53NO13/c38-35(39)9-11-41-13-15-43-17-19-45-21-23-47-25-27-49-28-26-48-24-22-46-20-18-44-16-14-42-12-10-37-36(40)50-29-34-32-7-3-1-5-30(32)31-6-2-4-8-33(31)34/h1-8,34H,9-29H2,(H,37,40)(H,38,39) | |
Source | PubChem | |
URL | https://pubchem.ncbi.nlm.nih.gov | |
Description | Data deposited in or computed by PubChem | |
InChI Key |
NEESTRVILHNOJT-UHFFFAOYSA-N | |
Source | PubChem | |
URL | https://pubchem.ncbi.nlm.nih.gov | |
Description | Data deposited in or computed by PubChem | |
Canonical SMILES |
C1=CC=C2C(=C1)C(C3=CC=CC=C32)COC(=O)NCCOCCOCCOCCOCCOCCOCCOCCOCCOCCC(=O)O | |
Source | PubChem | |
URL | https://pubchem.ncbi.nlm.nih.gov | |
Description | Data deposited in or computed by PubChem | |
Molecular Formula |
C36H53NO13 | |
Source | PubChem | |
URL | https://pubchem.ncbi.nlm.nih.gov | |
Description | Data deposited in or computed by PubChem | |
Molecular Weight |
707.8 g/mol | |
Source | PubChem | |
URL | https://pubchem.ncbi.nlm.nih.gov | |
Description | Data deposited in or computed by PubChem | |
Retrosynthesis Analysis
AI-Powered Synthesis Planning: Our tool employs the Template_relevance Pistachio, Template_relevance Bkms_metabolic, Template_relevance Pistachio_ringbreaker, Template_relevance Reaxys, Template_relevance Reaxys_biocatalysis model, leveraging a vast database of chemical reactions to predict feasible synthetic routes.
One-Step Synthesis Focus: Specifically designed for one-step synthesis, it provides concise and direct routes for your target compounds, streamlining the synthesis process.
Accurate Predictions: Utilizing the extensive PISTACHIO, BKMS_METABOLIC, PISTACHIO_RINGBREAKER, REAXYS, REAXYS_BIOCATALYSIS database, our tool offers high-accuracy predictions, reflecting the latest in chemical research and data.
Strategy Settings
Precursor scoring | Relevance Heuristic |
---|---|
Min. plausibility | 0.01 |
Model | Template_relevance |
Template Set | Pistachio/Bkms_metabolic/Pistachio_ringbreaker/Reaxys/Reaxys_biocatalysis |
Top-N result to add to graph | 6 |
Feasible Synthetic Routes
Disclaimer and Information on In-Vitro Research Products
Please be aware that all articles and product information presented on BenchChem are intended solely for informational purposes. The products available for purchase on BenchChem are specifically designed for in-vitro studies, which are conducted outside of living organisms. In-vitro studies, derived from the Latin term "in glass," involve experiments performed in controlled laboratory settings using cells or tissues. It is important to note that these products are not categorized as medicines or drugs, and they have not received approval from the FDA for the prevention, treatment, or cure of any medical condition, ailment, or disease. We must emphasize that any form of bodily introduction of these products into humans or animals is strictly prohibited by law. It is essential to adhere to these guidelines to ensure compliance with legal and ethical standards in research and experimentation.