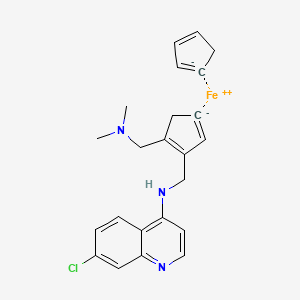
Ferroquine
- Click on QUICK INQUIRY to receive a quote from our team of experts.
- With the quality product at a COMPETITIVE price, you can focus more on your research.
Overview
Description
Ferroquine is a synthetic compound related to chloroquine, primarily known for its antimalarial properties. It is unique due to the presence of an organometallic ferrocene ring, which is uncommon in pharmaceuticals . This compound has shown significant activity against chloroquine-resistant strains of Plasmodium falciparum, the parasite responsible for the most severe form of malaria .
Mechanism of Action
Ferroquine, also known as SSR 97193 or FERROCHLOROQUINE, is a novel antimalarial drug candidate . It is the first organometallic drug, with a ferrocenyl group covalently flanked by a 4-aminoquinoline and a basic alkylamine .
Target of Action
This compound primarily targets the Plasmodium falciparum , the principal causative agent of malaria . It is designed to overcome the resistance problem of conventional drugs like chloroquine .
Mode of Action
This compound interacts with its targets by generating hydroxyl radicals, which break down the parasite’s digestive vacuole membrane . This action could be due to its ability to produce these radicals . Additionally, this compound’s action includes the production of HO (·) in the acidic digestive vacuole environment, inhibiting the reinvasion of the malaria parasite .
Biochemical Pathways
This compound affects multiple biochemical pathways. It has the capacity to target lipids, inhibit the formation of hemozoin, and generate reactive oxygen species . These actions contribute to its multifactorial mechanism of action .
Pharmacokinetics
The pharmacokinetic properties of this compound are still under investigation. It has been reported that this compound has reasonable safety and efficacy based on Phase II trials . .
Result of Action
The result of this compound’s action at the molecular and cellular level is the inhibition of the growth and proliferation of the malaria parasite. By generating hydroxyl radicals and breaking down the parasite’s digestive vacuole membrane, this compound prevents the parasite from reinvasion .
Action Environment
This compound is particularly effective in starved and hypoxic conditions frequently observed in advanced solid cancers . It negatively regulates Akt kinase and hypoxia-inducible factor-1α (HIF-1α), suggesting that environmental factors such as nutrient availability and oxygen levels can influence this compound’s action, efficacy, and stability .
Biochemical Analysis
Biochemical Properties
Ferroquine interacts with various enzymes and proteins in biochemical reactions. It targets lipids, inhibits the formation of hemozoin, and generates reactive oxygen species . Cytochrome P450 isoforms 2C9, 2C19, and 3A4, and possibly isoform 2D6 in some patients, are mainly involved in this compound oxidation .
Cellular Effects
This compound has significant effects on various types of cells and cellular processes. It potently inhibits autophagy, perturbs lysosomal function, and impairs tumor growth in vivo . This compound negatively regulates Akt kinase and hypoxia-inducible factor-1α(HIF-1α) and is particularly effective in starved and hypoxic conditions frequently observed in advanced solid cancers .
Molecular Mechanism
This compound exerts its effects at the molecular level through several mechanisms. It targets lipids, inhibits the formation of hemozoin, and generates reactive oxygen species . It also accumulates within and deacidifies lysosomes, induces lysosomal membrane permeabilization, mitochondrial depolarization, and caspase-independent cancer cell death .
Metabolic Pathways
This compound is involved in several metabolic pathways. It is metabolized mainly via an oxidative pathway into the major metabolite mono-N-demethyl this compound and then into di-N,N-demethyl this compound .
Subcellular Localization
One study showed that a ruthenocenic analogue of this compound accumulates faster in the parasitic digestive vacuole (DV) close to its membranes .
Preparation Methods
Ferroquine can be synthesized through a reductive amination process. The synthesis involves the condensation of aldehyde-amino ferrocene with 7-chloroquinoline-amine in the presence of a Lewis acid such as titanium isopropoxide, titanium tetrachloride, iron chloride, zinc chloride, aluminum chloride, or boron trifluoride . The condensation product is then reduced, followed by hydrolysis under ammonia or citric acid aqueous conditions .
Chemical Reactions Analysis
Ferroquine undergoes various chemical reactions, including:
Oxidation: this compound can be oxidized to form ferrocenium ions, which are reactive intermediates.
Reduction: The ferrocene moiety can be reduced back to its original state.
Substitution: The quinoline ring can undergo substitution reactions, particularly at the chloro position. Common reagents used in these reactions include oxidizing agents like ferric chloride and reducing agents like sodium borohydride.
Scientific Research Applications
Ferroquine has a wide range of scientific research applications:
Chemistry: It is used as a model compound to study organometallic chemistry and the behavior of ferrocene derivatives.
Biology: this compound is studied for its interactions with biological molecules and its potential as a bioorganometallic compound.
Medicine: Beyond its antimalarial properties, this compound is being investigated for its potential anticancer activity.
Comparison with Similar Compounds
Ferroquine is unique among antimalarial drugs due to its organometallic structure. Similar compounds include:
Chloroquine: The parent compound of this compound, used widely as an antimalarial but now less effective due to resistance.
Artemisinin: Another antimalarial with a different mechanism of action, often used in combination therapies.
Ferrocifens: Ferrocene analogs of tamoxifen, used in cancer treatment
Properties
CAS No. |
185055-67-8 |
---|---|
Molecular Formula |
C23H24ClFeN3 |
Molecular Weight |
433.8 g/mol |
IUPAC Name |
7-chloro-N-[[2-[(dimethylamino)methyl]cyclopenta-2,4-dien-1-yl]methyl]quinolin-4-amine;cyclopenta-1,3-diene;iron(2+) |
InChI |
InChI=1S/C18H19ClN3.C5H5.Fe/c1-22(2)12-14-5-3-4-13(14)11-21-17-8-9-20-18-10-15(19)6-7-16(17)18;1-2-4-5-3-1;/h3-10H,11-12H2,1-2H3,(H,20,21);1-5H;/q2*-1;+2 |
InChI Key |
DDENDDKMBDTHAX-UHFFFAOYSA-N |
SMILES |
CN(C)CC1=CC=C[C-]1CNC2=C3C=CC(=CC3=NC=C2)Cl.[CH-]1C=CC=C1.[Fe+2] |
Canonical SMILES |
CN(C)CC1=CC=C[C-]1CNC2=C3C=CC(=CC3=NC=C2)Cl.[CH-]1C=CC=C1.[Fe+2] |
Appearance |
Solid powder |
Purity |
>98% (or refer to the Certificate of Analysis) |
shelf_life |
>2 years if stored properly |
solubility |
Soluble in DMSO |
storage |
Dry, dark and at 0 - 4 C for short term (days to weeks) or -20 C for long term (months to years). |
Synonyms |
SR-97193; SSR-97193; SR97193; SSR97193; SR 97193; SSR 97193 |
Origin of Product |
United States |
Disclaimer and Information on In-Vitro Research Products
Please be aware that all articles and product information presented on BenchChem are intended solely for informational purposes. The products available for purchase on BenchChem are specifically designed for in-vitro studies, which are conducted outside of living organisms. In-vitro studies, derived from the Latin term "in glass," involve experiments performed in controlled laboratory settings using cells or tissues. It is important to note that these products are not categorized as medicines or drugs, and they have not received approval from the FDA for the prevention, treatment, or cure of any medical condition, ailment, or disease. We must emphasize that any form of bodily introduction of these products into humans or animals is strictly prohibited by law. It is essential to adhere to these guidelines to ensure compliance with legal and ethical standards in research and experimentation.