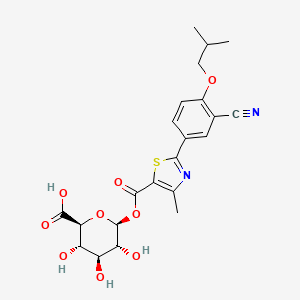
Febuxostat Acyl Glucuronide
Overview
Description
Febuxostat Acyl Glucuronide is a metabolite of Febuxostat, a non-purine selective inhibitor of xanthine oxidase. Febuxostat is primarily used to manage chronic hyperuricemia in patients with gout . The acyl glucuronide form is produced through the conjugation of Febuxostat with glucuronic acid, a process mediated by uridine diphosphate-glucuronosyltransferase enzymes .
Preparation Methods
Synthetic Routes and Reaction Conditions: The synthesis of Febuxostat Acyl Glucuronide involves the enzymatic conjugation of Febuxostat with glucuronic acid. This reaction is typically catalyzed by uridine diphosphate-glucuronosyltransferase enzymes such as UGT1A1, UGT1A3, UGT1A9, and UGT2B7 . The reaction conditions generally include an aqueous environment at physiological pH and temperature to facilitate the enzymatic activity.
Industrial Production Methods: Industrial production of this compound follows similar enzymatic processes but on a larger scale. The process involves the use of bioreactors to maintain optimal conditions for enzyme activity, ensuring efficient conversion of Febuxostat to its acyl glucuronide form. The product is then purified using chromatographic techniques to achieve the desired purity and concentration .
Chemical Reactions Analysis
Metabolic Formation Pathways
Febuxostat acyl glucuronide is generated through UGT-mediated conjugation , primarily involving:
- UGT1A1 , UGT1A3 , UGT1A9 , and UGT2B7 isoforms .
- This pathway accounts for 22–44% of febuxostat’s total metabolism , with the acyl glucuronide representing ~35% of the administered dose .
Key Reaction Steps:
- Glucuronidation : Febuxostat’s carboxylic acid group reacts with uridine diphosphate glucuronic acid (UDPGA), forming a β-1-O-acyl glucuronide bond .
- Competing Pathways : A smaller fraction undergoes oxidative metabolism via CYP enzymes (CYP1A2, CYP2C8, CYP2C9) .
Excretion and Elimination
This compound is eliminated through renal and hepatic pathways :
Parameter | Urine Excretion | Fecal Excretion |
---|---|---|
This compound | 30% of dose | 1% of dose |
Parent drug (unchanged) | 3% of dose | 12% of dose |
- Renal Clearance : Accounts for 49% of total elimination, with 30% attributed to the acyl glucuronide .
- Hydrolysis : The glucuronide may hydrolyze back to febuxostat in the bladder, contributing to urinary recovery of the parent drug .
Stability and Reactivity
Acyl glucuronides are prone to chemical degradation via:
- Intramolecular Acyl Migration : Forms positional isomers (β-2, β-3, β-4 glucuronides), which are resistant to enzymatic hydrolysis .
- Covalent Protein Binding : Reactive intermediates may form adducts with serum albumin or renal proteins, potentially contributing to hypersensitivity reactions .
Stability Data:
- Plasma Half-Life : this compound has a shorter half-life (~5–8 hours) compared to stable ether glucuronides .
- pH-Dependent Degradation : Accelerated in alkaline conditions, increasing isomerization risk .
Drug-Drug Interactions
This compound inhibits organic anion transporter 3 (OAT3) , altering the pharmacokinetics of co-administered drugs:
- Mechanism : Noncompetitive (febuxostat) and competitive (glucuronide) inhibition of OAT3-mediated transport .
Research Findings
Scientific Research Applications
Pharmacological Insights
Febuxostat acyl glucuronide is formed through the hepatic metabolism of febuxostat and plays a crucial role in its pharmacological profile. This metabolite exhibits potent inhibition of organic anion transporters, particularly Organic Anion Transporter 3 (OAT3), which has implications for drug-drug interactions, especially with medications such as rivaroxaban .
Table 1: Pharmacokinetic Properties of Febuxostat and Its Metabolites
Compound | Metabolism | Excretion | Key Features |
---|---|---|---|
Febuxostat | Hepatic (extensive) | Urine & feces | Non-purine xanthine oxidase inhibitor |
This compound | Glucuronidation | Urine (30%) | Potent OAT3 inhibitor; affects drug interactions |
Hydroxylated Metabolites | Various pathways | Urine & feces | Contributes to overall pharmacokinetics |
Clinical Applications
Febuxostat is primarily indicated for the treatment of gout and hyperuricemia. Clinical studies have demonstrated its efficacy in lowering serum uric acid levels more effectively than allopurinol, especially in patients with renal impairment .
Case Study: Long-term Efficacy of Febuxostat
A five-year study evaluated the long-term effects of febuxostat on patients with gout. The findings revealed that 93% of subjects maintained serum uric acid levels below 6.0 mg/dL, significantly reducing gout flare-ups . The study highlighted the sustained efficacy of febuxostat, even in patients who had previously not responded to allopurinol.
Safety Profile and Drug Interactions
While febuxostat is generally well-tolerated, its acyl glucuronide metabolite may contribute to adverse reactions due to its interaction with other drugs. Notably, the risk of cardiovascular events has been observed, particularly in patients with pre-existing conditions .
Table 2: Reported Adverse Reactions Associated with Febuxostat
Adverse Reaction | Incidence (%) |
---|---|
Liver function abnormalities | 3.5 |
Diarrhea | 2.7 |
Headache | 1.8 |
Nausea | 1.7 |
Rash | 1.5 |
Research Findings on Drug-Drug Interactions
Research indicates that this compound can influence the pharmacokinetics of co-administered drugs by inhibiting renal transporters. This interaction necessitates careful monitoring when prescribing febuxostat alongside other medications, particularly those that are substrates for OAT3 .
Mechanism of Action
The primary mechanism of action of Febuxostat Acyl Glucuronide involves its formation and subsequent clearance from the body. The conjugation with glucuronic acid enhances the solubility of Febuxostat, facilitating its excretion via the kidneys . The acyl glucuronide form can also interact with biological macromolecules through transacylation reactions, potentially influencing the pharmacokinetics and pharmacodynamics of Febuxostat .
Comparison with Similar Compounds
Allopurinol Acyl Glucuronide: Another xanthine oxidase inhibitor metabolite.
Diclofenac Acyl Glucuronide: A nonsteroidal anti-inflammatory drug metabolite.
Ibuprofen Acyl Glucuronide: A common analgesic and anti-inflammatory drug metabolite.
Uniqueness: Febuxostat Acyl Glucuronide is unique due to its selective inhibition of xanthine oxidase and its non-purine structure. Unlike purine analogs such as Allopurinol, Febuxostat and its metabolites do not interfere with purine metabolism, reducing the risk of certain side effects .
Biological Activity
Febuxostat, a selective xanthine oxidase inhibitor, is primarily used in the management of gout by lowering serum uric acid levels. Its major metabolite, Febuxostat Acyl Glucuronide (Febuxostat AG), plays a significant role in its pharmacological effects and interactions. This article explores the biological activity of Febuxostat AG, including its mechanisms of action, pharmacokinetics, and clinical implications.
Febuxostat inhibits both xanthine dehydrogenase (XDH) and xanthine oxidase (XO), enzymes involved in uric acid production. By doing so, it effectively reduces serum uric acid levels, which is crucial in treating gout. The acyl glucuronide metabolite also exhibits biological activity, contributing to the overall efficacy of febuxostat.
Key Mechanisms:
- Inhibition of Xanthine Oxidoreductase (XOR) : Febuxostat binds to the molybdenum-pterin active site of XOR, leading to decreased production of uric acid .
- Reduction of Reactive Oxygen Species (ROS) : By inhibiting both forms of XOR, febuxostat reduces oxidative stress and inflammation associated with gout flares .
Pharmacokinetics
Febuxostat is rapidly absorbed after oral administration, with about 85% bioavailability. It undergoes extensive hepatic metabolism primarily via UDP-glucuronosyltransferase (UGT) enzymes, producing Febuxostat AG as a significant metabolite.
Key Pharmacokinetic Parameters:
- Absorption : Tmax ranges from 1 to 1.5 hours post-administration.
- Metabolism : Approximately 22-44% of the dose is metabolized to Febuxostat AG .
- Elimination : About 49% of the dose is recovered in urine, with Febuxostat AG accounting for approximately 30% .
- Half-life : The terminal elimination half-life is approximately 5 to 8 hours .
Biological Activity and Drug Interactions
Recent studies have highlighted the role of Febuxostat AG in drug-drug interactions, particularly its inhibition of organic anion transporters such as OAT3. This inhibition can affect the pharmacokinetics of co-administered drugs.
Findings from Recent Research:
- OAT3 Inhibition : Both febuxostat and its acyl glucuronide metabolite significantly inhibit OAT3-mediated transport, which may lead to increased plasma concentrations of drugs that are substrates for this transporter .
- Clinical Implications : The inhibition of OAT3 suggests potential interactions with drugs like enalaprilat, necessitating careful monitoring when these medications are prescribed together .
Case Studies and Clinical Findings
A five-year study assessing the long-term efficacy and safety of febuxostat therapy in patients with gout demonstrated significant reductions in serum uric acid levels and a decrease in gout flares.
Key Results from Clinical Studies:
- Efficacy : 93% of subjects maintained serum uric acid levels below 6.0 mg/dL after five years .
- Gout Flare Resolution : Nearly complete resolution of gout flares was observed among participants who completed the study .
- Safety Profile : The treatment was generally well-tolerated, with mild to moderate adverse events reported .
Summary Table of Pharmacokinetic Parameters
Parameter | Value |
---|---|
Bioavailability | ~85% |
Tmax | 1 - 1.5 hours |
Metabolism | UGT enzymes (22-44%) |
Urinary Excretion | 49% (30% as Febuxostat AG) |
Half-life | 5 - 8 hours |
Q & A
Basic Research Questions
Q. What experimental methodologies are recommended to assess the chemical stability of Febuxostat Acyl Glucuronide in vitro?
To evaluate stability, incubate the glucuronide in 0.1 M phosphate buffer (pH 7.4) at 37°C, with timepoints analyzed via LC-MS/MS. Quench reactions using acetonitrile containing formic acid to stabilize isomers. Measure acyl migration (via peak multiplicity in LC-MS) and hydrolysis (aglycone formation). Stability assays should include glutathione/methoxylamine trapping to detect reactive intermediates (e.g., transacylation vs. glycation pathways) .
Q. Which UDP-glucuronosyltransferase (UGT) isoforms catalyze the formation of this compound?
Reaction phenotyping using recombinant UGT Supersomes® (e.g., UGT1A1, UGT1A3, UGT2B7) can identify isoforms involved. Incubate Febuxostat with individual UGT isoforms, quantify glucuronide formation via LC-MS, and compare activity across isoforms. Human liver microsomes (HLM) supplemented with alamethicin and saccharolactone are also used to mimic in vivo glucuronidation .
Q. How does acyl migration influence this compound’s reactivity and protein adduct formation?
Acyl migration generates positional isomers (2-β, 3-α, etc.), which can form covalent adducts with proteins via nucleophilic displacement. Use glutathione trapping in buffer to detect reactive transacylation intermediates. Methoxylamine trapping (for aldehyde intermediates) is less relevant, as glycation pathways are minimal compared to direct nucleophilic adduction .
Advanced Research Questions
Q. How can computational models predict the degradation kinetics of this compound?
Kinetic modeling tools like GEPASI or DFT calculations simulate transacylation and hydrolysis steps. Parameters include:
- Transacylation rate constants : Derived from NMR or LC-MS time-course data of isomerization.
- Activation energy (ΔE) : Calculated via DFT for transition states, correlated with experimental degradation rates.
For example, charged carboxylate groups in glucuronides stabilize transition states via intramolecular hydrogen bonding (C4-OH and aglycone), slowing degradation compared to neutral glucosides .
Q. How to resolve contradictions between in vitro reactivity data and in vivo toxicity findings for this compound?
Discrepancies arise due to:
- Systemic exposure : Low plasma levels in vivo may limit adduct formation despite high in vitro reactivity.
- Toxicokinetic thresholds : Use physiologically based pharmacokinetic (PBPK) modeling to correlate acyl glucuronide exposure with adduct detection in tissues.
- Clinical biomarkers : Monitor anti-adduct antibodies (via ELISA) and liver enzymes in trials to validate toxicity hypotheses .
Q. What advanced analytical techniques characterize acyl migration and hydrolysis pathways?
- NMR spectroscopy : Track anomeric proton shifts (δ 5.6–6.0 ppm for 1-β isomer) to distinguish isomers and quantify degradation routes.
- High-resolution MS/MS : Identify hydrolyzed aglycones and adducts (e.g., glutathione conjugates).
- Kinetic isotope effects (KIE) : Use deuterated buffers to probe reaction mechanisms (e.g., base-catalyzed hydrolysis) .
Q. How to predict the electrophilic potential of this compound’s transacylation reactions?
- Computational descriptors : Calculate ELUMO (energy of lowest unoccupied molecular orbital) and electrophilicity index for the transition state. ELUMO correlates strongly with degradation rates (r² = 0.90).
- Hydrogen bonding analysis : Intramolecular bonds (e.g., C4-OH to carboxylate in glucuronides) lower activation energy, increasing reactivity. Compare with glucosides lacking this stabilization .
Properties
IUPAC Name |
(2S,3S,4S,5R,6S)-6-[2-[3-cyano-4-(2-methylpropoxy)phenyl]-4-methyl-1,3-thiazole-5-carbonyl]oxy-3,4,5-trihydroxyoxane-2-carboxylic acid | |
---|---|---|
Source | PubChem | |
URL | https://pubchem.ncbi.nlm.nih.gov | |
Description | Data deposited in or computed by PubChem | |
InChI |
InChI=1S/C22H24N2O9S/c1-9(2)8-31-13-5-4-11(6-12(13)7-23)19-24-10(3)18(34-19)21(30)33-22-16(27)14(25)15(26)17(32-22)20(28)29/h4-6,9,14-17,22,25-27H,8H2,1-3H3,(H,28,29)/t14-,15-,16+,17-,22-/m0/s1 | |
Source | PubChem | |
URL | https://pubchem.ncbi.nlm.nih.gov | |
Description | Data deposited in or computed by PubChem | |
InChI Key |
ZRXRMGPMLDOOKN-FVMGUFKOSA-N | |
Source | PubChem | |
URL | https://pubchem.ncbi.nlm.nih.gov | |
Description | Data deposited in or computed by PubChem | |
Canonical SMILES |
CC1=C(SC(=N1)C2=CC(=C(C=C2)OCC(C)C)C#N)C(=O)OC3C(C(C(C(O3)C(=O)O)O)O)O | |
Source | PubChem | |
URL | https://pubchem.ncbi.nlm.nih.gov | |
Description | Data deposited in or computed by PubChem | |
Isomeric SMILES |
CC1=C(SC(=N1)C2=CC(=C(C=C2)OCC(C)C)C#N)C(=O)O[C@H]3[C@@H]([C@H]([C@@H]([C@H](O3)C(=O)O)O)O)O | |
Source | PubChem | |
URL | https://pubchem.ncbi.nlm.nih.gov | |
Description | Data deposited in or computed by PubChem | |
Molecular Formula |
C22H24N2O9S | |
Source | PubChem | |
URL | https://pubchem.ncbi.nlm.nih.gov | |
Description | Data deposited in or computed by PubChem | |
DSSTOX Substance ID |
DTXSID30747779 | |
Record name | 1-O-{2-[3-Cyano-4-(2-methylpropoxy)phenyl]-4-methyl-1,3-thiazole-5-carbonyl}-beta-D-glucopyranuronic acid | |
Source | EPA DSSTox | |
URL | https://comptox.epa.gov/dashboard/DTXSID30747779 | |
Description | DSSTox provides a high quality public chemistry resource for supporting improved predictive toxicology. | |
Molecular Weight |
492.5 g/mol | |
Source | PubChem | |
URL | https://pubchem.ncbi.nlm.nih.gov | |
Description | Data deposited in or computed by PubChem | |
CAS No. |
1351692-92-6 | |
Record name | 1-O-{2-[3-Cyano-4-(2-methylpropoxy)phenyl]-4-methyl-1,3-thiazole-5-carbonyl}-beta-D-glucopyranuronic acid | |
Source | EPA DSSTox | |
URL | https://comptox.epa.gov/dashboard/DTXSID30747779 | |
Description | DSSTox provides a high quality public chemistry resource for supporting improved predictive toxicology. | |
Disclaimer and Information on In-Vitro Research Products
Please be aware that all articles and product information presented on BenchChem are intended solely for informational purposes. The products available for purchase on BenchChem are specifically designed for in-vitro studies, which are conducted outside of living organisms. In-vitro studies, derived from the Latin term "in glass," involve experiments performed in controlled laboratory settings using cells or tissues. It is important to note that these products are not categorized as medicines or drugs, and they have not received approval from the FDA for the prevention, treatment, or cure of any medical condition, ailment, or disease. We must emphasize that any form of bodily introduction of these products into humans or animals is strictly prohibited by law. It is essential to adhere to these guidelines to ensure compliance with legal and ethical standards in research and experimentation.