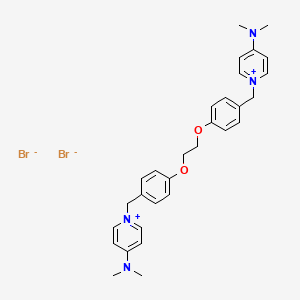
EB-3D
Overview
Description
EB-3D is a potent and selective inhibitor of choline kinase alpha 1 (ChoKα1), with an inhibitory concentration (IC50) of 1 micromolar. This compound has shown significant antiproliferative activity against various cancer cell lines, particularly T-leukemia cells . This compound affects choline kinase alpha expression, AMP-activated protein kinase (AMPK) activation, apoptosis, endoplasmic reticulum stress, and lipid metabolism, making it a promising candidate for cancer treatment .
Mechanism of Action
EB-3D, also known as “1,1’-(((Ethane-1,2-diylbis(oxy))bis(4,1-phenylene))bis(methylene))bis(4-(dimethylamino)pyridin-1-ium) bromide”, is a potent and selective choline kinase α (ChoKα) inhibitor . The following sections will detail its mechanism of action.
Target of Action
The primary target of this compound is choline kinase α (ChoKα) . This enzyme is the first step in the Kennedy pathway leading to the biosynthesis of phosphatidylcholine (PtdCho), the most abundant phospholipid in eukaryotic cell membranes . ChoKα is particularly overexpressed and hyperactivated in aggressive breast cancer .
Mode of Action
This compound interacts with its target, ChoKα, by inhibiting its activity . This results in a reduction in the synthesis of phosphocholine . Furthermore, this compound interferes with phosphatidylcholine biosynthesis via both the CDP-choline pathway and choline uptake by the cell .
Biochemical Pathways
The inhibition of ChoKα by this compound affects the phosphatidylcholine biosynthesis pathway . This disruption in phospholipid homeostasis is associated with an alteration in cholesterol metabolism and cell survival . The effects of this compound could be explained by the modulation of the AMP-activated protein kinase signaling pathway .
Result of Action
This compound has multifactorial effects including the inhibition of ChoKα enzyme activity, downregulation of ChoKα expression, AMPK activation, apoptosis dependent on ER stress, inhibition of basal autophagy, and deregulation of lipid metabolism . These effects lead to cellular senescence, reduced tumor growth, and metastatic dissemination in breast cancer .
Biochemical Analysis
Biochemical Properties
“EB-3D” exerts effects on ChoKα expression, AMPK activation, apoptosis, endoplasmic reticulum stress, and lipid metabolism . It has been demonstrated that “this compound” is able to reduce the synthesis of phosphocholine .
Cellular Effects
“this compound” strongly impairs breast cancer cell proliferation, migration, and invasion . It induces senescence in breast cancer cell lines through the activation of the metabolic sensor AMPK and the subsequent dephosphorylation of mTORC1 downstream targets .
Molecular Mechanism
The mechanism of action of “this compound” involves its binding to ChoKα, leading to inhibition of the enzyme and subsequent effects on cellular processes . This includes changes in gene expression, enzyme inhibition or activation, and changes in lipid metabolism .
Temporal Effects in Laboratory Settings
The effects of “this compound” over time in laboratory settings have not been fully elucidated. It has been shown to have potent antiproliferative activity in a panel of T-leukemia cell lines .
Metabolic Pathways
“this compound” is involved in the choline kinase pathway, where it inhibits the enzyme ChoKα . This leads to a reduction in the synthesis of phosphocholine .
Preparation Methods
Synthetic Routes and Reaction Conditions
The synthesis of EB-3D involves the preparation of symmetrical biscationic compounds. The process includes the following steps:
Formation of the core structure: The core structure of this compound is synthesized through a series of organic reactions, including nucleophilic substitution and condensation reactions.
Introduction of functional groups: Functional groups are introduced to the core structure to enhance its biological activity. This step involves the use of various reagents and catalysts under controlled conditions.
Purification and crystallization: The final product is purified using techniques such as column chromatography and recrystallization to achieve high purity.
Industrial Production Methods
Industrial production of this compound follows similar synthetic routes but on a larger scale. The process is optimized for efficiency and cost-effectiveness, ensuring high yield and purity. Key steps include:
Bulk synthesis: Large-scale reactors are used to carry out the chemical reactions, ensuring consistent quality and yield.
Purification: Advanced purification techniques, such as high-performance liquid chromatography (HPLC), are employed to remove impurities.
Quality control: Rigorous quality control measures are implemented to ensure the final product meets industry standards.
Chemical Reactions Analysis
Types of Reactions
EB-3D undergoes several types of chemical reactions, including:
Oxidation: this compound can be oxidized under specific conditions, leading to the formation of oxidized derivatives.
Reduction: The compound can also undergo reduction reactions, resulting in reduced forms with different biological activities.
Common Reagents and Conditions
Oxidation: Common oxidizing agents include hydrogen peroxide and potassium permanganate, typically used under acidic or basic conditions.
Reduction: Reducing agents such as sodium borohydride and lithium aluminum hydride are employed under controlled conditions.
Substitution: Various nucleophiles and electrophiles are used in substitution reactions, often in the presence of catalysts and solvents.
Major Products Formed
The major products formed from these reactions include oxidized, reduced, and substituted derivatives of this compound, each with distinct biological activities and potential therapeutic applications .
Scientific Research Applications
Antiproliferative Activity
The antiproliferative activity of EB-3D has been demonstrated across multiple cancer cell lines. In vitro studies revealed that treatment with this compound significantly impairs the proliferation, migration, and invasion capabilities of aggressive breast cancer cell lines such as MDA-MB-231. The compound also synergizes with conventional chemotherapeutic agents like doxorubicin, 5-fluorouracil, and cisplatin, enhancing their cytotoxic effects .
Table 1: Summary of Antiproliferative Effects of this compound
Cancer Cell Line | IC50 (µM) | Mechanism of Action |
---|---|---|
MDA-MB-231 | 0.31 | ChoKα1 inhibition leading to G1 phase arrest |
Other Lines | Varies | Reduction in phosphocholine synthesis |
Induction of Cellular Senescence
This compound has been shown to induce cellular senescence in breast cancer cells. This process is mediated through the activation of AMP-activated protein kinase (AMPK), which subsequently inhibits the mechanistic target of rapamycin complex 1 (mTORC1) signaling pathway. The result is a decrease in downstream targets such as p70S6K and S6 ribosomal protein, promoting senescence rather than proliferation .
In Vivo Studies
In vivo experiments using syngeneic orthotopic models have illustrated the efficacy of this compound in reducing tumor mass at low doses. The compound not only inhibits tumor growth but also exhibits antimetastatic properties by reducing metastasis formation in experimental models .
Table 2: In Vivo Effects of this compound
Study Type | Outcome | Dose (µM) |
---|---|---|
Tumor Growth Inhibition | Significant reduction | Low doses |
Metastasis Formation | Reduced metastasis | Low doses |
Case Studies
Several case studies have highlighted the potential clinical applications of this compound:
- Breast Cancer Treatment : A study demonstrated that combining this compound with standard chemotherapy significantly improved treatment outcomes in a mouse model of breast cancer.
- Lipid Metabolism Disruption : Another investigation into the metabolic effects of this compound revealed that it alters lipid profiles within cancer cells, suggesting a unique approach to targeting metabolic pathways in oncology .
Comparison with Similar Compounds
EB-3D is unique in its potent and selective inhibition of choline kinase alpha. Similar compounds include:
EB-3P: Another choline kinase alpha inhibitor with similar biological activities but different chemical structure.
Nevanimibe hydrochloride: An inhibitor of choline kinase with distinct molecular targets and pathways.
CP-724714: A compound with inhibitory effects on choline kinase but with different pharmacological properties.
This compound stands out due to its high selectivity and potency, making it a valuable tool in cancer research and potential therapeutic applications.
Biological Activity
EB-3D is a novel compound recognized for its potent biological activity, particularly as a choline kinase α1 (ChoKα1) inhibitor. This article explores its mechanisms of action, efficacy in cancer treatment, and potential therapeutic applications based on diverse research findings.
This compound targets ChoKα1, an enzyme critical in the biosynthesis of phosphatidylcholine, a major component of cell membranes. By inhibiting ChoKα1, this compound reduces phosphocholine levels, which leads to:
- Cellular Senescence : this compound induces senescence in breast cancer cells through the activation of the AMP-activated protein kinase (AMPK) pathway. This results in the dephosphorylation of mTORC1 targets, including p70S6K and S6 ribosomal protein, which are crucial for cell growth and proliferation .
- Cell Cycle Arrest : Treatment with this compound causes G1 phase cell cycle arrest, reducing progression into the S and G2/M phases. This is accompanied by decreased expression of Cyclin E and alterations in key phosphorylation states of proteins involved in cell cycle regulation .
In Vitro Studies
This compound exhibits significant antiproliferative effects against various breast cancer cell lines, including MDA-MB-231, MDA-MB-468, and MCF-7. The compound has been shown to:
- Impair Proliferation : After 24 hours of treatment with 1 µM this compound, there was a notable increase in free choline levels (212.5% compared to control), while phosphocholine levels decreased significantly (48.9% after 48 hours) .
- Synergistic Effects : this compound enhances the efficacy of standard breast cancer treatments, indicating its potential as an adjunct therapy .
In Vivo Studies
The antitumor effects of this compound were evaluated using the E0771 mouse model of breast cancer. Key findings include:
- Tumor Reduction : At low doses, this compound significantly reduced tumor mass.
- Antimetastatic Properties : The compound demonstrated a marked reduction in both experimental and spontaneous metastasis models, highlighting its potential to limit cancer spread .
Research Findings Summary
The following table summarizes key findings from various studies on the biological activity of this compound:
Study Type | Key Findings |
---|---|
In Vitro | - Induces cellular senescence via AMPK activation. - Causes G1 phase arrest in cell cycle. - Reduces phosphocholine levels significantly. |
In Vivo | - Significant tumor mass reduction in E0771 mouse model. - Demonstrates antimetastatic effects. |
Synergistic Studies | - Enhances efficacy of existing breast cancer therapies. |
Case Studies
Recent studies have highlighted the promising role of this compound in clinical settings:
- Breast Cancer Treatment : A case study involving multiple breast cancer cell lines indicated that this compound not only inhibits proliferation but also enhances the effectiveness of conventional chemotherapeutics.
- Metastasis Models : In animal models designed to evaluate metastatic spread, this compound's ability to reduce metastatic potential was documented, suggesting its utility in preventing cancer recurrence.
Properties
IUPAC Name |
1-[[4-[2-[4-[[4-(dimethylamino)pyridin-1-ium-1-yl]methyl]phenoxy]ethoxy]phenyl]methyl]-N,N-dimethylpyridin-1-ium-4-amine;dibromide | |
---|---|---|
Source | PubChem | |
URL | https://pubchem.ncbi.nlm.nih.gov | |
Description | Data deposited in or computed by PubChem | |
InChI |
InChI=1S/C30H36N4O2.2BrH/c1-31(2)27-13-17-33(18-14-27)23-25-5-9-29(10-6-25)35-21-22-36-30-11-7-26(8-12-30)24-34-19-15-28(16-20-34)32(3)4;;/h5-20H,21-24H2,1-4H3;2*1H/q+2;;/p-2 | |
Source | PubChem | |
URL | https://pubchem.ncbi.nlm.nih.gov | |
Description | Data deposited in or computed by PubChem | |
InChI Key |
SCXVGPSPZQBBDM-UHFFFAOYSA-L | |
Source | PubChem | |
URL | https://pubchem.ncbi.nlm.nih.gov | |
Description | Data deposited in or computed by PubChem | |
Canonical SMILES |
CN(C)C1=CC=[N+](C=C1)CC2=CC=C(C=C2)OCCOC3=CC=C(C=C3)C[N+]4=CC=C(C=C4)N(C)C.[Br-].[Br-] | |
Source | PubChem | |
URL | https://pubchem.ncbi.nlm.nih.gov | |
Description | Data deposited in or computed by PubChem | |
Molecular Formula |
C30H36Br2N4O2 | |
Source | PubChem | |
URL | https://pubchem.ncbi.nlm.nih.gov | |
Description | Data deposited in or computed by PubChem | |
Molecular Weight |
644.4 g/mol | |
Source | PubChem | |
URL | https://pubchem.ncbi.nlm.nih.gov | |
Description | Data deposited in or computed by PubChem | |
Retrosynthesis Analysis
AI-Powered Synthesis Planning: Our tool employs the Template_relevance Pistachio, Template_relevance Bkms_metabolic, Template_relevance Pistachio_ringbreaker, Template_relevance Reaxys, Template_relevance Reaxys_biocatalysis model, leveraging a vast database of chemical reactions to predict feasible synthetic routes.
One-Step Synthesis Focus: Specifically designed for one-step synthesis, it provides concise and direct routes for your target compounds, streamlining the synthesis process.
Accurate Predictions: Utilizing the extensive PISTACHIO, BKMS_METABOLIC, PISTACHIO_RINGBREAKER, REAXYS, REAXYS_BIOCATALYSIS database, our tool offers high-accuracy predictions, reflecting the latest in chemical research and data.
Strategy Settings
Precursor scoring | Relevance Heuristic |
---|---|
Min. plausibility | 0.01 |
Model | Template_relevance |
Template Set | Pistachio/Bkms_metabolic/Pistachio_ringbreaker/Reaxys/Reaxys_biocatalysis |
Top-N result to add to graph | 6 |
Feasible Synthetic Routes
Disclaimer and Information on In-Vitro Research Products
Please be aware that all articles and product information presented on BenchChem are intended solely for informational purposes. The products available for purchase on BenchChem are specifically designed for in-vitro studies, which are conducted outside of living organisms. In-vitro studies, derived from the Latin term "in glass," involve experiments performed in controlled laboratory settings using cells or tissues. It is important to note that these products are not categorized as medicines or drugs, and they have not received approval from the FDA for the prevention, treatment, or cure of any medical condition, ailment, or disease. We must emphasize that any form of bodily introduction of these products into humans or animals is strictly prohibited by law. It is essential to adhere to these guidelines to ensure compliance with legal and ethical standards in research and experimentation.