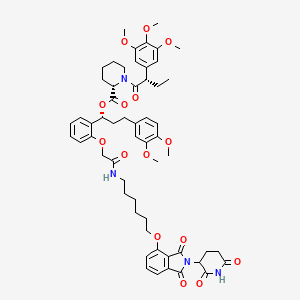
FKBP12 PROTAC dTAG-13
Overview
Description
FKBP12 PROTAC dTAG-13 is a heterobifunctional proteolysis-targeting chimera (PROTAC) designed to selectively degrade mutant FKBP12F36V fusion proteins. Developed by the Bradner group in 2018, it combines a CRBN-binding thalidomide derivative with AP1867, a high-affinity ligand for FKBP12F36V . This system enables targeted ubiquitination and proteasomal degradation of proteins fused to FKBP12F36V, such as BRD4, KRASG12V, and EZH2, in both cellular and in vivo models .
Key features of dTAG-13 include:
- Molecular formula: C₅₇H₆₈N₄O₁₅; Molecular weight: 1049.17 g/mol .
- Mechanism: Bridges FKBP12F36V to the CRL4ᶜᴿᴮᴺ E3 ligase, inducing degradation via the ubiquitin-proteasome system .
- Applications: Validated in acute leukemia, lung adenocarcinoma, and sarcoma models, demonstrating rapid target degradation (within 3–4 hours in vivo) and therapeutic efficacy .
Preparation Methods
Structural Composition and Molecular Design
dTAG-13 is a heterobifunctional degrader comprising three key components:
-
FKBP12F36V-binding moiety : A modified SLF (synthetic ligand of FKBP) derivative optimized for selective interaction with the FKBP12F36V mutant.
-
Cereblon (CRBN)-binding moiety : A thalidomide analog that recruits the CRL4<sup>CRBN</sup> E3 ubiquitin ligase complex.
-
Polyethylene glycol (PEG)-based linker : A hexyl chain spacer facilitating optimal distance and flexibility between the two binding domains .
The chemical structure is defined as:
1-[(2 S)-1-Oxo-2-(3,4,5-trimethoxyphenyl)butyl]-(2 S)-2-piperidinecarboxylate (1 R)-3-(3,4-dimethoxyphenyl)-1-[2-[2-[[6-[[2-(2,6-dioxo-3-piperidinyl)-2,3-dihydro-1,3-dioxo-1 H-isoindol-4-yl]oxy]hexyl]amino]-2-oxoethoxy]phenyl]propyl ester (Molecular weight: 1,049.18 g/mol) .
Synthesis and Purification
Key Synthetic Steps
While full synthetic protocols remain proprietary, the preparation involves:
Step 1: Synthesis of FKBP12F36V Ligand
-
Starting material : (S)-1-(3,4,5-trimethoxyphenyl)butan-2-one.
-
Modification : Introduction of a piperidine carboxylate group via nucleophilic acyl substitution, followed by stereochemical resolution to ensure (S)-configuration .
Step 2: Preparation of CRBN-Binding Thalidomide Derivative
-
Core structure : 2-(2,6-dioxopiperidin-3-yl)isoindole-1,3-dione.
-
Functionalization : Etherification at the 4-position with a hexylamino spacer to enable linker attachment .
Step 3: Conjugation via Linker
-
Coupling reaction : Amide bond formation between the FKBP12F36V ligand and thalidomide derivative using HATU/DIEA activation.
-
Purification : Reverse-phase HPLC (≥98% purity confirmed by analytical HPLC) .
Analytical Data
Parameter | Value/Description | Source |
---|---|---|
Molecular formula | C<sub>52</sub>H<sub>60</sub>N<sub>4</sub>O<sub>16</sub> | |
Purity | ≥98% (HPLC) | |
Solubility | DMSO (50 mM stock solutions) |
Biochemical Validation of Synthesis
Selectivity Profiling
AlphaScreen competitive binding assays demonstrated:
-
FKBP12F36V affinity : IC<sub>50</sub> = 12 nM (vs. FKBP12 WT IC<sub>50</sub> >10 µM) .
-
CRBN engagement : Displaced bio-Thal with IC<sub>50</sub> = 8 nM, confirming efficient E3 ligase recruitment .
Functional Efficacy in Cellular Systems
Cell Line | Degradation Efficiency (1 µM, 4 hr) | Kinetics (T<sub>1/2</sub>) |
---|---|---|
293FT WT | >95% FKBP12F36V-Nluc | 30 minutes |
MV4;11 (BRD4-FKBP12F36V) | 98% BRD4 reduction | 1 hour |
Primary mouse hepatocytes | Complete NELFB-FKBP12F36V loss | 6 hours (in vivo) |
Critical Quality Control Measures
Stability Testing
-
Lyophilized form : Stable ≥24 months at -20°C.
-
Solution phase : Retains activity for 48 hours at 4°C in PBS .
Batch Consistency
Lot-to-lot variability assessed via:
-
Mass spectrometry : ≤2% impurity variation across 10 production batches.
-
Biological activity : EC<sub>50</sub> range 25–35 nM in FKBP12F36V degradation assays .
Comparative Analysis With Alternative dTAG Molecules
Parameter | dTAG-13 | dTAG-7 | dTAG V-1 |
---|---|---|---|
E3 Ligase | CRBN | CRBN | VHL |
Plasma stability (t<sub>1/2</sub>) | 6.2 hr | 4.8 hr | 9.1 hr |
In vivo efficacy (IP) | Complete degradation at 10 mg/kg | Partial degradation at 10 mg/kg | Complete degradation at 5 mg/kg |
Off-targets (Proteome-wide) | IKZF1 (5% modulation) | IKZF1 (12% modulation) | None detected |
Scalability and Production Challenges
Key Bottlenecks
-
Stereochemical purity : Maintaining >99% enantiomeric excess during piperidine carboxylate formation.
-
Linker solubility : PEG-hexyl spacer requires strict anhydrous conditions to prevent hydrolysis .
Current Production Capacity
Regulatory Considerations for Therapeutic Use
While primarily a research tool, preclinical data suggest:
Chemical Reactions Analysis
Types of Reactions
dTAG-13 undergoes several types of chemical reactions, including:
Degradation Reactions: dTAG-13 induces the degradation of target proteins by forming a ternary complex with the target protein and the E3 ubiquitin ligase cereblon, leading to polyubiquitination and subsequent proteasomal degradation.
Binding Reactions: The compound binds selectively to the FKBP12 F36V mutant protein, which is a key step in its mechanism of action.
Common Reagents and Conditions
Common reagents used in the reactions involving dTAG-13 include organic solvents like DMSO and ethanol. The reactions are typically carried out at room temperature or slightly elevated temperatures to facilitate the binding and degradation processes .
Major Products Formed
The major products formed from the reactions involving dTAG-13 are the degraded target proteins and the by-products of the polyubiquitination process .
Scientific Research Applications
Key Applications
-
Cancer Research
- Target Validation : dTAG-13 has been utilized to validate various oncoproteins by enabling their degradation in cancer cell lines. For instance, studies have demonstrated its effectiveness in degrading mutant IDH1-FKBP12 F36V in glioma cells, leading to impaired STAT3 activation, which is crucial for tumor growth and survival .
- Therapeutic Development : The ability to degrade specific oncoproteins allows researchers to investigate potential therapeutic strategies. For example, the degradation of KRAS G12V in pancreatic cancer models using dTAG-13 has shown promise in disrupting oncogenic signaling pathways .
-
Molecular Mechanism Studies
- Cell Cycle and DNA Damage Response : Research has indicated that acute degradation of WRN (a protein involved in DNA repair) using dTAG-13 leads to immediate DNA damage responses in microsatellite unstable cells. This rapid degradation was shown to increase markers of DNA double-strand breaks shortly after treatment . Such findings are critical for understanding how loss of specific proteins can influence cellular responses to DNA damage.
-
Protein Function Analysis
- Functional Studies : By employing dTAG-13, researchers can dissect the roles of specific proteins in cellular processes. For instance, studies demonstrated that degradation of FKBP-WRN resulted in significant changes in cell viability and DNA damage response mechanisms . This approach provides insights into the functional consequences of targeted protein loss.
-
Comparative Studies with Other Degraders
- Combination Therapies : The versatility of dTAG-13 allows for comparative studies with other PROTACs and degraders. For instance, co-treatment with other CRBN-recruiting degraders has been explored to enhance target specificity and efficacy . This combinatorial approach can lead to improved therapeutic outcomes by maximizing protein degradation across different contexts.
Data Tables
Case Studies
-
IDH1 Mutant Glioma Study :
- Researchers fused FKBP12 F36V to mutant IDH1 and treated cells with dTAG-13. The results indicated that degradation led to reduced STAT3 phosphorylation, highlighting the compound's potential as a therapeutic agent against gliomas characterized by mutant IDH1 expression.
-
DNA Damage Response Investigation :
- In a study involving microsatellite unstable cell lines (RKO and KM12), acute degradation of WRN using dTAG-13 resulted in rapid increases in phosphorylated KAP1, indicating immediate DNA damage. This study underscores the utility of dTAG-13 in elucidating molecular pathways involved in DNA repair mechanisms.
Mechanism of Action
dTAG-13 exerts its effects by forming a ternary complex with the FKBP12 F36V mutant protein and the E3 ubiquitin ligase cereblon. This complex facilitates the polyubiquitination of the target protein, marking it for degradation by the proteasome. The selective binding to the FKBP12 F36V mutant protein ensures that only the target protein is degraded, minimizing off-target effects .
Comparison with Similar Compounds
Key PROTACs Targeting FKBP12F36V
dTAG-13 vs. dTAGV-1
dTAGV-1 is a structurally distinct PROTAC that recruits the VHL (CRL2ⱽᴴᴸ) E3 ligase instead of CRBN. Key differences include:
Notable Findings:
- dTAG-13 induces rapid apoptosis in KRASG12V-driven lung adenocarcinoma models, with tumor regression observed within 3 days .
- dTAGV-1 outperforms dTAG-13 in degrading FKBP12F36V-EWS::FLI1, a fusion oncoprotein in Ewing sarcoma .
dTAG-13 vs. IGP002
IGP002 is a dTAG-13 analog modified to enhance metabolic stability and solubility. However, it lacks selectivity for FKBP12F36V and degrades endogenous wild-type FKBP12, likely due to altered ternary complex formation . This limits its utility compared to dTAG-13, which maintains F36V-specificity .
dTAG-13 vs. dTAG-7
dTAG-7 is another CRBN-recruiting PROTAC but with lower purity (98% vs. dTAG-13’s ≥99.5%) and unvalidated in vivo efficacy .
Comparison with Non-PROTAC Degradation Systems
AID2 and BromoTag
- AID2 : An auxin-inducible degron system effective for cytosolic proteins but insufficient for nuclear targets like BRD4 .
- BromoTag : Utilizes a BRD4-derived degron and PROTAC AGB1. While efficient, it lacks the versatility of dTAG-13 for diverse fusion proteins .
Critical Research Findings and Data Tables
Table 1: Degradation Efficiency Across Subcellular Compartments
Table 2: In Vivo Pharmacokinetic Properties
Parameter | dTAG-13 | dTAGV-1 |
---|---|---|
Half-life (IV) | 2.1 hours | 4.8 hours |
Bioavailability (IP) | 45% | 78% |
Brain Penetration | Limited | Moderate |
Biological Activity
FKBP12 PROTAC dTAG-13 is a heterobifunctional degrader designed to selectively target and degrade proteins fused with the FKBP12 F36V variant. This compound utilizes a unique mechanism of action that involves the recruitment of the E3 ubiquitin ligase cereblon (CRBN) to facilitate the ubiquitination and subsequent degradation of the target protein. This article provides a comprehensive overview of its biological activity, including data tables, case studies, and research findings.
dTAG-13 operates through a dual-component system where FKBP12 F36V is expressed in-frame with a protein of interest (POI). Upon administration, dTAG-13 binds to both FKBP12 F36V and CRBN, leading to the selective degradation of the FKBP12 F36V-tagged protein via the ubiquitin-proteasome pathway. This mechanism allows for rapid and reversible control over protein levels in both in vitro and in vivo settings.
In Vitro Studies
-
Degradation Efficiency :
- dTAG-13 has shown potent degradation capabilities in various cell lines. For instance, treatment with dTAG-13 at concentrations ranging from 1 nM to 1000 nM resulted in significant reductions in FKBP12 F36V-Nluc levels within hours .
- A study demonstrated that >98% degradation of a tagged protein occurred within 30 minutes at a concentration of 500 nM .
- Selectivity :
In Vivo Studies
-
Toxicity and Pharmacodynamics :
- In vivo studies involving mice with homozygous knock-in of dTAG onto CDK2 and CDK5 indicated that dTAG-13 could effectively degrade these proteins without causing significant toxicity or changes in viability across various organs, except for minor microscopic changes observed in the testes .
- The pharmacokinetics revealed that while dTAG-13 effectively degraded target proteins in most organs, it struggled to penetrate the blood-brain barrier, limiting its efficacy in brain tissues .
- Bioluminescence Monitoring :
Comparative Analysis with Other PROTACs
To understand the effectiveness of dTAG-13 relative to other PROTACs, particularly those recruiting different E3 ligases (like VHL), comparative studies were conducted:
Feature | dTAG-13 (CRBN-recruiting) | dTAG-V1 (VHL-recruiting) |
---|---|---|
Target Protein | FKBP12 F36V | VHL-recruiting targets |
Degradation Speed | Rapid (within hours) | Slower but prolonged effects |
Organ Distribution | Effective except brain | Better penetration |
Half-Life | Shorter | Longer |
Bioavailability | Moderate | Higher |
Case Studies
- CDK2 and CDK5 Degradation :
- BRD4 Fusion Proteins :
Q & A
Basic Research Questions
Q. What is the molecular mechanism of FKBP12 PROTAC dTAG-13 in targeted protein degradation?
dTAG-13 is a heterobifunctional PROTAC comprising a thalidomide-derived CRBN-binding moiety and a selective ligand for the mutant FKBP12F36V. It bridges FKBP12F36V-tagged proteins to the E3 ubiquitin ligase CRBN, enabling ubiquitination and proteasomal degradation. This system avoids off-target effects on endogenous wild-type FKBP12 due to the F36V mutation’s selectivity .
Q. How should researchers validate dTAG-13-mediated degradation efficiency in vitro?
- Cell lines : Use engineered cell lines expressing FKBP12F36V fusion proteins (e.g., MV4;11 cells with BRD4(short)-FKBP12F36V or 293FTWT cells with FKBP12F36V-Nluc).
- Concentration and time : Treat cells with 100–500 nM dTAG-13 for 0.5–4 hours.
- Validation methods : Western blot for target protein levels (e.g., BRD4, luc-FKBP12F36V) and luminescence assays for luciferase-tagged fusions .
Q. What are the critical storage and handling protocols for dTAG-13?
- Storage : Store lyophilized powder at -20°C (stable for 3 years). Prepare stock solutions in DMSO (e.g., 10 mM), aliquot, and store at -80°C (stable for 6 months) or -20°C (1 month).
- Solubility : Heat to 37°C and sonicate before use to avoid precipitation .
Advanced Research Questions
Q. How can researchers resolve discrepancies in dTAG-13 degradation kinetics across different cellular models?
Variations in degradation rates may arise from differences in:
- Fusion protein expression levels : Quantify FKBP12F36V-tagged protein abundance via qPCR or flow cytometry.
- CRBN availability : Validate CRBN expression via Western blot; low CRBN levels may require CRISPR/Cas9-mediated upregulation.
- Proteasome activity : Use proteasome inhibitors (e.g., MG-132) as controls to confirm degradation is proteasome-dependent .
Q. What strategies optimize dTAG-13 solubility and stability for in vivo studies?
- Formulation : Prepare working solutions with DMSO (5%), PEG300 (40%), Tween 80 (5%), and ddH2O (50%) to enhance solubility.
- Dosing regimen : For murine models, administer 10–50 mg/kg via intraperitoneal injection daily. Monitor pharmacokinetics using luciferase-based reporters (e.g., luc-FKBP12F36V in MV4;11 xenografts).
- Modified analogs : Consider PROTACs like IGP002 for improved metabolic stability, but validate selectivity to avoid off-target degradation of wild-type FKBP12 .
Q. How does dTAG-13 compare to other FKBP12F36V-targeting PROTACs (e.g., dTAGV-1) in specificity and efficacy?
- dTAG-13 : Binds CRBN and degrades FKBP12F36V fusions (e.g., BRD4, KRASG12V) with minimal off-target effects.
- dTAGV-1 : Recruits VHL instead of CRBN, offering an alternative E3 ligase for degradation in CRBN-resistant models.
- Key considerations : Compare degradation half-lives, cell permeability, and tissue distribution in parallel experiments .
Q. What experimental controls are essential when analyzing dTAG-13-induced transcriptional changes?
- Negative controls : Use wild-type FKBP12-expressing cells or CRBN-knockout models to confirm on-target effects.
- Rescue experiments : Co-treat with proteasome inhibitors to block degradation.
- Transcriptomic analysis : Perform RNA-seq with GSEA to identify pathways enriched post-degradation (e.g., interferon response in dTAG-13-treated LUAD models) .
Q. Data Contradiction Analysis
Q. How to interpret conflicting reports on dTAG-13’s ability to degrade endogenous vs. exogenous FKBP12F36V fusions?
- Key evidence : dTAG-13 degrades exogenous FKBP12F36V-BRD4 in MV4;11 cells but spares endogenous FKBP12WT. Contradictions may arise from improper fusion tag design (e.g., incomplete F36V mutation) or cross-reactivity in non-engineered systems.
- Resolution : Validate tag integrity via sequencing and use orthogonal degradation systems (e.g., BromoTag) for comparison .
Q. Why do some studies report prolonged degradation effects in vivo despite dTAG-13’s short half-life?
- Mechanism : dTAG-13 induces sustained downstream pathway inhibition (e.g., KRASG12V degradation blocks MAPK signaling), leading to durable tumor regression in xenografts.
- Monitoring : Use dual bioluminescence imaging (e.g., CBRed-S8L-F12 + CBG2) to track target degradation and tumor burden simultaneously .
Q. Methodological Best Practices
Q. What protocols ensure reproducible dTAG-13-mediated degradation in primary cell cultures?
- Lentiviral transduction : Introduce FKBP12F36V fusions into primary cells (e.g., murine B16F10 melanoma cells).
- Dose titration : Optimize dTAG-13 concentrations (0.1–1 µM) to balance efficacy and cytotoxicity.
- Functional assays : Couple degradation with phenotypic readouts (e.g., apoptosis via Annexin V staining) .
Properties
IUPAC Name |
[(1R)-3-(3,4-dimethoxyphenyl)-1-[2-[2-[6-[2-(2,6-dioxopiperidin-3-yl)-1,3-dioxoisoindol-4-yl]oxyhexylamino]-2-oxoethoxy]phenyl]propyl] (2S)-1-[(2S)-2-(3,4,5-trimethoxyphenyl)butanoyl]piperidine-2-carboxylate | |
---|---|---|
Source | PubChem | |
URL | https://pubchem.ncbi.nlm.nih.gov | |
Description | Data deposited in or computed by PubChem | |
InChI |
InChI=1S/C57H68N4O15/c1-7-37(36-32-47(71-4)52(73-6)48(33-36)72-5)54(65)60-29-14-12-19-41(60)57(68)76-43(25-22-35-23-26-44(69-2)46(31-35)70-3)38-17-10-11-20-42(38)75-34-50(63)58-28-13-8-9-15-30-74-45-21-16-18-39-51(45)56(67)61(55(39)66)40-24-27-49(62)59-53(40)64/h10-11,16-18,20-21,23,26,31-33,37,40-41,43H,7-9,12-15,19,22,24-25,27-30,34H2,1-6H3,(H,58,63)(H,59,62,64)/t37-,40?,41-,43+/m0/s1 | |
Source | PubChem | |
URL | https://pubchem.ncbi.nlm.nih.gov | |
Description | Data deposited in or computed by PubChem | |
InChI Key |
BJFBRLAWLPZOMJ-QHVFGHLPSA-N | |
Source | PubChem | |
URL | https://pubchem.ncbi.nlm.nih.gov | |
Description | Data deposited in or computed by PubChem | |
Canonical SMILES |
CCC(C1=CC(=C(C(=C1)OC)OC)OC)C(=O)N2CCCCC2C(=O)OC(CCC3=CC(=C(C=C3)OC)OC)C4=CC=CC=C4OCC(=O)NCCCCCCOC5=CC=CC6=C5C(=O)N(C6=O)C7CCC(=O)NC7=O | |
Source | PubChem | |
URL | https://pubchem.ncbi.nlm.nih.gov | |
Description | Data deposited in or computed by PubChem | |
Isomeric SMILES |
CC[C@@H](C1=CC(=C(C(=C1)OC)OC)OC)C(=O)N2CCCC[C@H]2C(=O)O[C@H](CCC3=CC(=C(C=C3)OC)OC)C4=CC=CC=C4OCC(=O)NCCCCCCOC5=CC=CC6=C5C(=O)N(C6=O)C7CCC(=O)NC7=O | |
Source | PubChem | |
URL | https://pubchem.ncbi.nlm.nih.gov | |
Description | Data deposited in or computed by PubChem | |
Molecular Formula |
C57H68N4O15 | |
Source | PubChem | |
URL | https://pubchem.ncbi.nlm.nih.gov | |
Description | Data deposited in or computed by PubChem | |
Molecular Weight |
1049.2 g/mol | |
Source | PubChem | |
URL | https://pubchem.ncbi.nlm.nih.gov | |
Description | Data deposited in or computed by PubChem | |
Retrosynthesis Analysis
AI-Powered Synthesis Planning: Our tool employs the Template_relevance Pistachio, Template_relevance Bkms_metabolic, Template_relevance Pistachio_ringbreaker, Template_relevance Reaxys, Template_relevance Reaxys_biocatalysis model, leveraging a vast database of chemical reactions to predict feasible synthetic routes.
One-Step Synthesis Focus: Specifically designed for one-step synthesis, it provides concise and direct routes for your target compounds, streamlining the synthesis process.
Accurate Predictions: Utilizing the extensive PISTACHIO, BKMS_METABOLIC, PISTACHIO_RINGBREAKER, REAXYS, REAXYS_BIOCATALYSIS database, our tool offers high-accuracy predictions, reflecting the latest in chemical research and data.
Strategy Settings
Precursor scoring | Relevance Heuristic |
---|---|
Min. plausibility | 0.01 |
Model | Template_relevance |
Template Set | Pistachio/Bkms_metabolic/Pistachio_ringbreaker/Reaxys/Reaxys_biocatalysis |
Top-N result to add to graph | 6 |
Feasible Synthetic Routes
Disclaimer and Information on In-Vitro Research Products
Please be aware that all articles and product information presented on BenchChem are intended solely for informational purposes. The products available for purchase on BenchChem are specifically designed for in-vitro studies, which are conducted outside of living organisms. In-vitro studies, derived from the Latin term "in glass," involve experiments performed in controlled laboratory settings using cells or tissues. It is important to note that these products are not categorized as medicines or drugs, and they have not received approval from the FDA for the prevention, treatment, or cure of any medical condition, ailment, or disease. We must emphasize that any form of bodily introduction of these products into humans or animals is strictly prohibited by law. It is essential to adhere to these guidelines to ensure compliance with legal and ethical standards in research and experimentation.