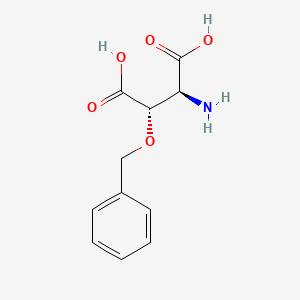
DL-TBOA
Overview
Description
DL-Threo-β-Benzyloxyaspartic acid, commonly known as DL-TBOA, is a potent inhibitor of excitatory amino acid transporters (EAATs). It is widely used in scientific research to study the role of glutamate transporters in various physiological and pathological processes. This compound is known for its ability to block the uptake of glutamate, an important neurotransmitter in the central nervous system, thereby influencing synaptic transmission and neuronal excitability .
Preparation Methods
Synthetic Routes and Reaction Conditions
DL-TBOA is synthesized through a multi-step process involving the protection and deprotection of functional groups, as well as the formation of key intermediatesThe final step involves the deprotection of the amino group to yield this compound .
Industrial Production Methods
While specific industrial production methods for this compound are not widely documented, the compound is generally produced in specialized chemical laboratories under controlled conditions. The synthesis involves the use of high-purity reagents and solvents, as well as advanced purification techniques to ensure the final product meets the required specifications .
Chemical Reactions Analysis
Types of Reactions
DL-TBOA primarily undergoes substitution reactions due to the presence of reactive functional groups such as the amino and carboxyl groups. It can also participate in esterification and amidation reactions .
Common Reagents and Conditions
Common reagents used in the synthesis and modification of this compound include protecting agents like tert-butyloxycarbonyl (Boc) and benzyl chloroformate, as well as deprotecting agents like trifluoroacetic acid. The reactions are typically carried out under mild conditions to prevent degradation of the compound .
Major Products Formed
The major products formed from the reactions involving this compound include various protected and deprotected intermediates, as well as derivatives with modified functional groups. These products are often used in further studies to explore the structure-activity relationships of the compound .
Scientific Research Applications
DL-TBOA has a wide range of applications in scientific research, particularly in the fields of neuroscience, pharmacology, and cancer research. Some of its key applications include:
Neuroscience: this compound is used to study the role of glutamate transporters in synaptic transmission and neuronal excitability. .
Pharmacology: The compound is used to investigate the effects of glutamate transporter inhibition on drug-induced neurotoxicity and to develop potential therapeutic strategies for conditions like epilepsy and ischemia
Cancer Research: this compound has been shown to influence the viability of cancer cells, particularly in colorectal cancer. .
Mechanism of Action
DL-TBOA exerts its effects by competitively inhibiting excitatory amino acid transporters, specifically EAAT1, EAAT2, and EAAT3. By blocking the uptake of glutamate, this compound increases the extracellular concentration of this neurotransmitter, leading to enhanced synaptic transmission and neuronal excitability. This mechanism is particularly useful in studying the role of glutamate transporters in various physiological and pathological processes .
Comparison with Similar Compounds
DL-TBOA is often compared with other glutamate transporter inhibitors such as L-trans-pyrrolidine-2,4-dicarboxylate (t-2,4-PDC). While both compounds inhibit glutamate uptake, this compound is more potent and selective for EAATs. Unlike t-2,4-PDC, this compound is not transported by the glutamate carriers, making it a more effective tool for studying the role of glutamate transporters in synaptic transmission .
Similar Compounds
L-trans-Pyrrolidine-2,4-Dicarboxylate (t-2,4-PDC): A substrate inhibitor of glutamate transporters, less potent than this compound.
Dihydrokainate: A selective inhibitor of EAAT2, less potent than this compound.
UCPH-101: A selective inhibitor of EAAT1, used in studies to differentiate the roles of various EAAT subtypes.
This compound’s unique properties and high potency make it a valuable tool in scientific research, particularly in the study of glutamate transporters and their role in various physiological and pathological processes.
Biological Activity
DL-Threo-β-Benzyloxyaspartic acid (DL-TBOA) is a potent inhibitor of excitatory amino acid transporters (EAATs), which play a critical role in regulating extracellular levels of glutamate, a key neurotransmitter in the central nervous system. This article explores the biological activity of this compound, focusing on its mechanisms, effects on cell viability, and potential neurotoxic and neuroprotective properties.
This compound acts primarily as a competitive inhibitor of EAATs, particularly EAAT1 and EAAT2. The compound has been shown to inhibit glutamate uptake in various cellular models:
- In vitro Studies : In COS-1 cells overexpressing EAAT1, this compound exhibited an inhibition constant (Ki) of 42 µM. For EAAT2, the Ki was significantly lower at 5.7 µM, indicating a stronger inhibitory effect compared to other known blockers like dihydrokainate (Ki = 79 µM) .
- In vivo Studies : Administration of this compound via microdialysis into the hippocampus of rats resulted in a substantial increase in extracellular levels of both aspartate (3.4-fold) and glutamate (9-fold), demonstrating its efficacy in altering neurotransmitter dynamics .
Effects on Cell Viability
This compound's impact on cell viability has been extensively studied in cancer cell lines. Research indicates that this compound can have dual effects depending on the context:
- Cancer Cell Lines : In studies involving HCT116 and LoVo cells, this compound enhanced cell death induced by SN38 (an active metabolite of irinotecan) while counteracting cell death caused by oxaliplatin. Specifically, treatment with 350 µM this compound abolished oxaliplatin-induced loss of viability in LoVo cells . This suggests that this compound may modulate resistance mechanisms in cancer therapy.
- Mechanistic Insights : The differential effects observed are linked to changes in the expression and localization of SLC1A1, an important glutamate transporter. In resistant cell lines, this compound treatment led to increased sensitivity to SN38 while providing protection against oxaliplatin-induced toxicity .
Neurotoxic and Neuroprotective Properties
This compound's role extends beyond cancer biology; it also exhibits neurotoxic and neuroprotective effects:
- Neurotoxicity : High doses of this compound have been associated with neuronal damage in the hippocampus, evidenced by convulsive behavior and limbic seizures in animal models following stereotaxic administration . This underscores the potential risks associated with excessive inhibition of glutamate transporters.
- Neuroprotection : Conversely, under certain conditions, this compound may provide neuroprotective effects by preventing excitotoxicity associated with elevated glutamate levels. The balance between its neurotoxic and neuroprotective roles is critical for understanding its therapeutic potential .
Summary of Findings
The biological activity of this compound is characterized by its potent inhibition of EAATs, leading to significant alterations in glutamate dynamics and cellular viability across various contexts. Below is a summary table encapsulating key findings:
Property | Value/Observation |
---|---|
EAAT1 Ki | 42 µM |
EAAT2 Ki | 5.7 µM |
Effect on SN38-induced death | Enhanced by this compound |
Effect on oxaliplatin-induced death | Counteracted by this compound |
Neurotoxicity | Induces neuronal damage at high doses |
Neuroprotection | Potentially protective under certain conditions |
Properties
IUPAC Name |
(2S,3S)-2-amino-3-phenylmethoxybutanedioic acid | |
---|---|---|
Source | PubChem | |
URL | https://pubchem.ncbi.nlm.nih.gov | |
Description | Data deposited in or computed by PubChem | |
InChI |
InChI=1S/C11H13NO5/c12-8(10(13)14)9(11(15)16)17-6-7-4-2-1-3-5-7/h1-5,8-9H,6,12H2,(H,13,14)(H,15,16)/t8-,9-/m0/s1 | |
Source | PubChem | |
URL | https://pubchem.ncbi.nlm.nih.gov | |
Description | Data deposited in or computed by PubChem | |
InChI Key |
BYOBCYXURWDEDS-IUCAKERBSA-N | |
Source | PubChem | |
URL | https://pubchem.ncbi.nlm.nih.gov | |
Description | Data deposited in or computed by PubChem | |
Canonical SMILES |
C1=CC=C(C=C1)COC(C(C(=O)O)N)C(=O)O | |
Source | PubChem | |
URL | https://pubchem.ncbi.nlm.nih.gov | |
Description | Data deposited in or computed by PubChem | |
Isomeric SMILES |
C1=CC=C(C=C1)CO[C@@H]([C@@H](C(=O)O)N)C(=O)O | |
Source | PubChem | |
URL | https://pubchem.ncbi.nlm.nih.gov | |
Description | Data deposited in or computed by PubChem | |
Molecular Formula |
C11H13NO5 | |
Source | PubChem | |
URL | https://pubchem.ncbi.nlm.nih.gov | |
Description | Data deposited in or computed by PubChem | |
DSSTOX Substance ID |
DTXSID60415515 | |
Record name | (2S,3S)-2-amino-3-phenylmethoxybutanedioic acid | |
Source | EPA DSSTox | |
URL | https://comptox.epa.gov/dashboard/DTXSID60415515 | |
Description | DSSTox provides a high quality public chemistry resource for supporting improved predictive toxicology. | |
Molecular Weight |
239.22 g/mol | |
Source | PubChem | |
URL | https://pubchem.ncbi.nlm.nih.gov | |
Description | Data deposited in or computed by PubChem | |
CAS No. |
208706-75-6 | |
Record name | (2S,3S)-2-amino-3-phenylmethoxybutanedioic acid | |
Source | EPA DSSTox | |
URL | https://comptox.epa.gov/dashboard/DTXSID60415515 | |
Description | DSSTox provides a high quality public chemistry resource for supporting improved predictive toxicology. | |
Q1: What is the primary target of DL-TBOA?
A1: this compound primarily targets excitatory amino acid transporters (EAATs) []. These transporters play a crucial role in regulating extracellular glutamate levels in the central nervous system by transporting it into surrounding glial cells, thereby terminating glutamatergic synaptic transmission.
Q2: How does this compound interact with EAATs?
A2: this compound acts as a competitive, non-transported blocker of EAATs []. It binds to the transporter's substrate binding site, preventing glutamate from binding and being transported. Unlike some inhibitors, this compound does not induce transporter-mediated currents or heteroexchange with intracellular glutamate [].
Q3: What are the downstream effects of inhibiting EAATs with this compound?
A3: Inhibiting EAATs with this compound leads to an accumulation of glutamate in the extracellular space [, ]. This can have various consequences, including:
- Enhanced activation of extrasynaptic glutamate receptors: Elevated glutamate levels can lead to prolonged activation of both synaptic and extrasynaptic NMDA and AMPA receptors, contributing to neuronal excitability and potentially excitotoxicity [, ].
- Modulation of synaptic plasticity: this compound has been shown to affect both long-term potentiation (LTP) and long-term depression (LTD) in the hippocampus, highlighting its influence on synaptic plasticity mechanisms [, ].
- Altered neuronal network activity: By disrupting glutamate homeostasis, this compound can induce abnormal neuronal activity patterns, such as slow network oscillations (SNOs) in the developing hippocampus and paroxysmal hyperactivity in the prefrontal cortex of AD mouse models [, ].
Q4: What is the molecular formula and weight of this compound?
A4: The molecular formula of this compound is C12H15NO5, and its molecular weight is 253.25 g/mol. Detailed spectroscopic data can be found in relevant chemical databases and publications.
Q5: Is this compound chemically stable, and how does its stability compare to other EAAT inhibitors?
A5: this compound demonstrates higher chemical stability compared to its benzoyl analog, Threo-Beta-Benzoyloxyaspartate (TBzOAsp) []. This improved stability is attributed to the replacement of the labile ester bond in TBzOAsp with a more stable ether linkage in this compound.
Q6: Does this compound have any known catalytic properties?
A6: this compound is not known to possess any catalytic properties. Its primary mode of action is through binding and inhibiting EAATs, not catalyzing chemical reactions.
Q7: Have computational methods been used to study this compound and its interactions with EAATs?
A7: Yes, computational chemistry and modeling techniques, including molecular docking, have been employed to investigate the binding interactions between this compound and EAATs []. These studies help elucidate the structural basis of this compound's inhibitory potency and selectivity.
Q8: How do structural modifications of this compound affect its activity and selectivity for different EAAT subtypes?
A8: Research on novel L-TBOA analogs with substitutions on the benzene ring has shown that these modifications significantly influence their potency and selectivity for EAAT subtypes []. For instance, TFB-TBOA, with a trifluoromethylbenzoylamino group, displayed significantly higher potency for EAAT1 and EAAT2 compared to L-TBOA []. These findings underscore the importance of SAR studies in optimizing this compound derivatives for targeted therapeutic applications.
Disclaimer and Information on In-Vitro Research Products
Please be aware that all articles and product information presented on BenchChem are intended solely for informational purposes. The products available for purchase on BenchChem are specifically designed for in-vitro studies, which are conducted outside of living organisms. In-vitro studies, derived from the Latin term "in glass," involve experiments performed in controlled laboratory settings using cells or tissues. It is important to note that these products are not categorized as medicines or drugs, and they have not received approval from the FDA for the prevention, treatment, or cure of any medical condition, ailment, or disease. We must emphasize that any form of bodily introduction of these products into humans or animals is strictly prohibited by law. It is essential to adhere to these guidelines to ensure compliance with legal and ethical standards in research and experimentation.