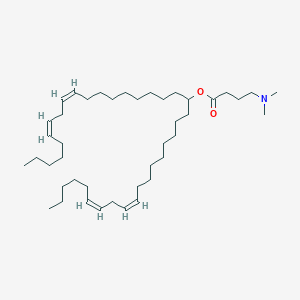
D-Lin-MC3-DMA
Overview
Description
Mechanism of Action
Target of Action
D-Lin-MC3-DMA, also known as DLin-MC3-DMA or Q0J6FQ6FKP, is an ionizable cationic lipid . It is primarily used in the formation of lipid nanoparticles (LNPs) for delivering cargos such as oligonucleotides into living cells . The primary targets of this compound are hepatocytes and hepatic stellate cells (HSCs), which are particularly attractive targets for RNA therapy .
Mode of Action
This compound encapsulates the negatively charged RNA cargos and facilitates their cytosolic transport . This is achieved through the unique pH-dependent charge variability of this compound. Under acidic conditions, it exhibits a positive charge, allowing it to bind and protect the RNA cargo. At physiological pH, it becomes electrically neutral, minimizing cytotoxicity .
Biochemical Pathways
The biochemical pathways affected by this compound primarily involve the delivery of RNA cargos to target cells. For instance, this compound is used in siRNA therapy to knock down specific proteins in hepatocytes . The delivered siRNA can then interact with the cell’s machinery to inhibit the expression of the target protein, affecting the associated biochemical pathways .
Pharmacokinetics
The pharmacokinetic properties of this compound are largely determined by its formulation into LNPs. These nanoparticles are designed to protect the RNA cargo during delivery and facilitate its release into the cytoplasm of target cells . .
Result of Action
The result of this compound action is the effective delivery of RNA cargos to target cells, leading to the desired gene silencing effects. For example, this compound has been used to deliver siRNA that knocks down coagulation factor VII (FVII) in hepatocytes and ADAMTS13 in HSCs . This leads to a reduction in the levels of these proteins, demonstrating the effectiveness of this compound in RNA delivery .
Action Environment
The action of this compound can be influenced by the delivery route. For instance, a study found that the immune response to an mRNA vaccine encapsulated in this compound varied depending on whether it was administered via the intramuscular or subcutaneous route . This suggests that the environment in which this compound operates can affect its efficacy and stability .
Biochemical Analysis
Biochemical Properties
D-Lin-MC3-DMA plays a pivotal role in biochemical reactions by forming complexes with negatively charged nucleic acids such as siRNA and mRNA. This interaction is facilitated by the ionizable nature of this compound, which allows it to adopt a positive charge at acidic pH and a neutral charge at physiological pH . This pH-dependent charge variability enables this compound to effectively bind to nucleic acids and protect them from degradation by nucleases. Additionally, this compound interacts with various proteins and enzymes involved in the endosomal escape of nucleic acids, thereby enhancing their delivery to the cytoplasm .
Cellular Effects
This compound has profound effects on various types of cells and cellular processes. It influences cell function by facilitating the delivery of nucleic acids into the cytoplasm, where they can exert their therapeutic effects. This compound has been shown to impact cell signaling pathways, gene expression, and cellular metabolism by enabling the efficient delivery of siRNA and mRNA . For instance, this compound-containing LNPs have been used to deliver siRNA targeting specific genes, leading to gene silencing and subsequent changes in cellular function .
Molecular Mechanism
The mechanism of action of this compound involves several key steps. Upon administration, this compound forms complexes with nucleic acids, protecting them from degradation. These complexes are then taken up by cells through endocytosis. Within the acidic environment of endosomes, this compound becomes positively charged, facilitating the escape of nucleic acids into the cytoplasm . This endosomal escape is crucial for the therapeutic efficacy of nucleic acids, as it allows them to reach their target sites within the cell. Additionally, this compound can modulate gene expression by delivering mRNA that encodes therapeutic proteins .
Temporal Effects in Laboratory Settings
In laboratory settings, the effects of this compound have been studied over time to assess its stability, degradation, and long-term impact on cellular function. Studies have shown that this compound-containing LNPs exhibit good stability and can protect nucleic acids from degradation for extended periods . The long-term effects of this compound on cellular function are still being investigated, with some studies indicating potential changes in cellular metabolism and gene expression over time .
Dosage Effects in Animal Models
The effects of this compound vary with different dosages in animal models. At lower doses, this compound-containing LNPs have been shown to effectively deliver nucleic acids and achieve therapeutic effects without significant toxicity . At higher doses, there may be threshold effects and potential toxic or adverse effects, including changes in liver function and immune responses . These findings highlight the importance of optimizing dosage to achieve the desired therapeutic outcomes while minimizing adverse effects.
Metabolic Pathways
This compound is involved in several metabolic pathways, particularly those related to the delivery and processing of nucleic acids. It interacts with enzymes and cofactors that facilitate the endosomal escape and cytoplasmic release of nucleic acids . Additionally, this compound can influence metabolic flux and metabolite levels by modulating gene expression and cellular metabolism . These interactions are crucial for the efficient delivery and therapeutic efficacy of nucleic acids.
Transport and Distribution
Within cells and tissues, this compound is transported and distributed through various mechanisms. It interacts with transporters and binding proteins that facilitate its uptake and localization within cells . Once inside the cell, this compound-containing LNPs can accumulate in specific tissues, such as the liver, where they release their nucleic acid cargo . This targeted distribution enhances the therapeutic efficacy of nucleic acids while minimizing off-target effects.
Subcellular Localization
The subcellular localization of this compound is critical for its activity and function. After endocytosis, this compound-containing LNPs localize to endosomes, where the acidic environment triggers the release of nucleic acids into the cytoplasm . This release is facilitated by the pH-dependent charge variability of this compound, which allows it to interact with endosomal membranes and promote nucleic acid escape . Additionally, this compound may undergo post-translational modifications that direct it to specific cellular compartments, further enhancing its therapeutic potential .
Preparation Methods
Synthetic Routes and Reaction Conditions
The synthesis of D-Lin-MC3-DMA involves multiple steps, starting with the preparation of the core lipid structure. The process typically includes the esterification of 4-(N,N-dimethylamino) butyric acid with a long-chain unsaturated alcohol. The reaction conditions often require the use of catalysts and specific temperature controls to ensure the desired product is obtained .
Industrial Production Methods
In industrial settings, the production of this compound is scaled up using optimized synthetic routes that ensure high yield and purity. The process involves continuous monitoring and adjustment of reaction parameters to maintain consistency. Advanced purification techniques such as chromatography are employed to isolate the final product .
Chemical Reactions Analysis
Types of Reactions
D-Lin-MC3-DMA undergoes various chemical reactions, including:
Oxidation: This reaction can modify the lipid structure, potentially affecting its ionizable properties.
Reduction: This reaction can be used to alter the functional groups on the lipid, impacting its interaction with nucleic acids.
Common Reagents and Conditions
Common reagents used in these reactions include oxidizing agents like hydrogen peroxide, reducing agents such as sodium borohydride, and various catalysts to facilitate substitution reactions. The conditions typically involve controlled temperatures and pH levels to ensure the reactions proceed efficiently .
Major Products Formed
The major products formed from these reactions depend on the specific reagents and conditions used. For example, oxidation may result in the formation of hydroxylated derivatives, while substitution reactions can introduce new functional groups that enhance the lipid’s ability to form stable nanoparticles .
Scientific Research Applications
D-Lin-MC3-DMA has a wide range of scientific research applications:
Comparison with Similar Compounds
Similar Compounds
Uniqueness
This compound stands out due to its low toxicity and high efficiency in delivering nucleic acids. Compared to ALC-0315 and SM-102, this compound has shown superior performance in gene silencing applications, particularly in liver-targeted therapies . Its unique pH-dependent charge properties allow for efficient encapsulation and release of nucleic acids, making it a preferred choice for many therapeutic applications .
Properties
IUPAC Name |
[(6Z,9Z,28Z,31Z)-heptatriaconta-6,9,28,31-tetraen-19-yl] 4-(dimethylamino)butanoate | |
---|---|---|
Source | PubChem | |
URL | https://pubchem.ncbi.nlm.nih.gov | |
Description | Data deposited in or computed by PubChem | |
InChI |
InChI=1S/C43H79NO2/c1-5-7-9-11-13-15-17-19-21-23-25-27-29-31-33-35-38-42(46-43(45)40-37-41-44(3)4)39-36-34-32-30-28-26-24-22-20-18-16-14-12-10-8-6-2/h13-16,19-22,42H,5-12,17-18,23-41H2,1-4H3/b15-13-,16-14-,21-19-,22-20- | |
Source | PubChem | |
URL | https://pubchem.ncbi.nlm.nih.gov | |
Description | Data deposited in or computed by PubChem | |
InChI Key |
NRLNQCOGCKAESA-KWXKLSQISA-N | |
Source | PubChem | |
URL | https://pubchem.ncbi.nlm.nih.gov | |
Description | Data deposited in or computed by PubChem | |
Canonical SMILES |
CCCCCC=CCC=CCCCCCCCCC(CCCCCCCCC=CCC=CCCCCC)OC(=O)CCCN(C)C | |
Source | PubChem | |
URL | https://pubchem.ncbi.nlm.nih.gov | |
Description | Data deposited in or computed by PubChem | |
Isomeric SMILES |
CCCCC/C=C\C/C=C\CCCCCCCCC(OC(=O)CCCN(C)C)CCCCCCCC/C=C\C/C=C\CCCCC | |
Source | PubChem | |
URL | https://pubchem.ncbi.nlm.nih.gov | |
Description | Data deposited in or computed by PubChem | |
Molecular Formula |
C43H79NO2 | |
Source | PubChem | |
URL | https://pubchem.ncbi.nlm.nih.gov | |
Description | Data deposited in or computed by PubChem | |
Molecular Weight |
642.1 g/mol | |
Source | PubChem | |
URL | https://pubchem.ncbi.nlm.nih.gov | |
Description | Data deposited in or computed by PubChem | |
CAS No. |
1224606-06-7 | |
Record name | Dlin-mc3-dma | |
Source | ChemIDplus | |
URL | https://pubchem.ncbi.nlm.nih.gov/substance/?source=chemidplus&sourceid=1224606067 | |
Description | ChemIDplus is a free, web search system that provides access to the structure and nomenclature authority files used for the identification of chemical substances cited in National Library of Medicine (NLM) databases, including the TOXNET system. | |
Record name | MC-3 | |
Source | FDA Global Substance Registration System (GSRS) | |
URL | https://gsrs.ncats.nih.gov/ginas/app/beta/substances/Q0J6FQ6FKP | |
Description | The FDA Global Substance Registration System (GSRS) enables the efficient and accurate exchange of information on what substances are in regulated products. Instead of relying on names, which vary across regulatory domains, countries, and regions, the GSRS knowledge base makes it possible for substances to be defined by standardized, scientific descriptions. | |
Explanation | Unless otherwise noted, the contents of the FDA website (www.fda.gov), both text and graphics, are not copyrighted. They are in the public domain and may be republished, reprinted and otherwise used freely by anyone without the need to obtain permission from FDA. Credit to the U.S. Food and Drug Administration as the source is appreciated but not required. | |
ANone: While the exact fate is not fully elucidated, research suggests that after releasing the nucleic acid cargo, DLin-MC3-DMA likely integrates into cellular membranes or undergoes degradation and clearance. [, ]
ANone: Unfortunately, the exact molecular formula and weight are not consistently reported in the provided research papers.
ANone: Studies show that DLin-MC3-DMA-based LNPs can exhibit varying stability depending on factors like temperature, pH, and buffer composition. [, ] Research indicates that buffers like Tris and HEPES provide better cryoprotection than PBS. []
ANone: DLin-MC3-DMA-based LNPs have shown promise in various applications, including siRNA therapy, mRNA vaccines, and gene editing. [, , , , , ] Notably, it is a key component of the FDA-approved siRNA therapy, Onpattro®. [, ]
ANone: DLin-MC3-DMA is not typically considered a catalyst and its catalytic properties are not explored in the provided research.
ANone: Molecular Dynamics (MD) simulations have been extensively employed to study the behavior of DLin-MC3-DMA in lipid bilayers, its interaction with other LNP components like helper lipids, and its role in membrane fusion. [, , , , ]
ANone: While the research doesn't explicitly mention QSAR models specifically for DLin-MC3-DMA, structure-activity relationship studies have investigated the impact of modifications on lipid properties and LNP efficacy. [, , ]
ANone: Research indicates that alterations in the headgroup, linker, and tail regions of DLin-MC3-DMA can significantly impact LNP properties such as transfection efficiency, biodistribution, and toxicity. [, , , ] For instance, incorporating a disulfide bond in the linker region can enhance endosomal escape and mRNA delivery. []
ANone: Lipid shape, particularly the balance between the headgroup and tail regions, plays a critical role in LNP formation, stability, and intracellular trafficking. [] The charge state of DLin-MC3-DMA, influenced by pH and its shape, affects its interaction with other lipids and nucleic acids. []
ANone: Research suggests optimization of buffer composition, lipid ratios, and the addition of cryoprotectants like sucrose can improve LNP stability during storage. []
ANone: The choice and ratio of helper lipids like cholesterol, DSPC, and PEGylated lipids greatly influence LNP size, charge, encapsulation efficiency, and in vivo behavior. [, , , , , , ]
ANone: While the provided research focuses on the scientific aspects, it doesn't delve into specific SHE regulations for DLin-MC3-DMA. It is crucial to consult relevant regulatory guidelines for manufacturing and handling.
ANone: Studies on patisiran, an FDA-approved siRNA therapy using DLin-MC3-DMA, show that it exhibits a two-phase PK profile with a relatively long terminal elimination half-life. [, ]
ANone: Various cell lines, including hepatocytes (HuH-7) and immune cells, have been employed to assess LNP uptake, endosomal escape, gene silencing efficiency, and protein expression. [, , , , , ]
ANone: Rodent models, particularly mice, are frequently utilized to investigate LNP biodistribution, therapeutic efficacy, and potential toxicity. [, , , , ] Non-human primate studies have also been conducted to assess safety and efficacy. []
ANone: The provided research does not extensively discuss resistance mechanisms specific to DLin-MC3-DMA.
ANone: While generally well-tolerated, research suggests that high doses of DLin-MC3-DMA-based LNPs can lead to transient liver enzyme elevations in some cases. [, , ]
ANone: Long-term safety data is still being gathered, but current research indicates that DLin-MC3-DMA-based LNPs have a manageable safety profile. []
ANone: Research is exploring surface modifications with targeting ligands like peptides, antibodies, or aptamers to enhance LNP specificity to tissues beyond the liver. [, , ]
ANone: Yes, studies have investigated alternative routes like intramuscular and subcutaneous administration, particularly for mRNA vaccines, with varying success in achieving target gene expression. [, , , ]
ANone: The research primarily focuses on the delivery aspects, with limited discussion on specific biomarkers for DLin-MC3-DMA-based therapies.
ANone: Common techniques include dynamic light scattering (DLS) for size and polydispersity index (PDI), transmission electron microscopy (TEM) for morphology, and zeta potential measurements for surface charge. [, , , , ]
ANone: Techniques like fluorescence spectroscopy or gel electrophoresis are often used to quantify encapsulated nucleic acids and determine encapsulation efficiency. [, , ]
ANone: The provided research primarily focuses on the biomedical applications and doesn't delve into details regarding environmental impact, recycling, alternatives, or historical context.
ANone: The development and understanding of DLin-MC3-DMA-based therapies require expertise from various fields, including:
- Chemistry: Synthesis and characterization of novel lipids. [, , , ]
- Biochemistry: Understanding lipid-nucleic acid interactions and intracellular trafficking pathways. [, , ]
- Pharmaceutics: Formulation optimization for enhanced stability, delivery, and targeting. [, , , ]
- Immunology: Elucidating the immune responses elicited by LNP-based vaccines and therapeutics. [, ]
Disclaimer and Information on In-Vitro Research Products
Please be aware that all articles and product information presented on BenchChem are intended solely for informational purposes. The products available for purchase on BenchChem are specifically designed for in-vitro studies, which are conducted outside of living organisms. In-vitro studies, derived from the Latin term "in glass," involve experiments performed in controlled laboratory settings using cells or tissues. It is important to note that these products are not categorized as medicines or drugs, and they have not received approval from the FDA for the prevention, treatment, or cure of any medical condition, ailment, or disease. We must emphasize that any form of bodily introduction of these products into humans or animals is strictly prohibited by law. It is essential to adhere to these guidelines to ensure compliance with legal and ethical standards in research and experimentation.