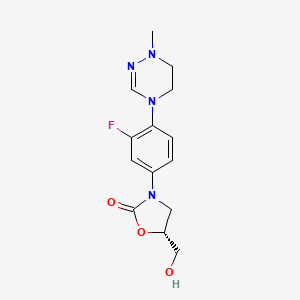
Delpazolid
Overview
Description
Mechanism of Action
Target of Action
Delpazolid, also known as LCB01-0371, is an antibiotic that primarily targets Gram-positive bacteria , including methicillin-resistant Staphylococcus aureus (MRSA), vancomycin-resistant enterococci (VRE), and Mycobacterium tuberculosis . These bacteria are responsible for a range of infections, including drug-resistant tuberculosis (MDR-TB).
Mode of Action
This compound belongs to the class of antimicrobials known as oxazolidinones . Oxazolidinones act by inhibiting protein synthesis in bacteria . They bind to the bacterial ribosome, preventing the formation of a functional 70S-initiation complex, which is an essential component of the protein synthesis process .
Biochemical Pathways
The primary biochemical pathway affected by this compound is the protein synthesis pathway in bacteria . By inhibiting this pathway, this compound prevents bacteria from producing essential proteins, which leads to a cessation of bacterial growth and eventually bacterial death .
Pharmacokinetics
The pharmacokinetics of this compound have been evaluated in clinical trials . It has been found to have excellent human bioavailability, with a bioavailability of approximately 100% . This means it can be administered orally or intravenously . This compound also has a low protein binding rate (37% in humans), which means a larger proportion of the drug remains free in the bloodstream to exert its therapeutic effect . It is cleared rapidly from the blood, reducing the risk of toxicity .
Result of Action
The primary result of this compound’s action is the inhibition of bacterial growth . By preventing bacteria from synthesizing proteins, this compound effectively halts bacterial replication. This results in a reduction in the bacterial population, helping to clear the infection . In the case of MDR-TB, this compound has been shown to significantly reduce resistance rates compared to linezolid .
Biochemical Analysis
Biochemical Properties
Delpazolid acts by inhibiting protein synthesis, a mechanism similar to other oxazolidinones . This inhibition of protein synthesis is intended to kill bacteria, but it can also impair mitochondria inside eukaryotic cells . In vitro studies have shown that this compound has similar or better activity compared to Linezolid against most Gram-positive bacteria and mycobacteria .
Cellular Effects
This compound has been shown to have inhibitory effects on mitochondrial protein synthesis in human cells, similar to those of linezolid . It can improve the minimum bactericidal concentration of Mycobacterium tuberculosis H37Rv and significantly reduce resistance rates, especially of multi-drug-resistant tuberculosis (MDR-TB) isolates .
Molecular Mechanism
The underlying antibacterial mechanism of this compound is similar to that of oxazolidinone in that it inhibits bacterial protein synthesis, which kills or inhibits the growth of bacteria . Protein synthesis also occurs in the mitochondria of eukaryotes, although mitochondria use independent protein-synthesis machinery that differs from that of bacteria .
Temporal Effects in Laboratory Settings
This compound has been evaluated in a phase 1 clinical trial, which revealed that it does not cause adverse events such as myelosuppression even after three weeks of repeated dosing . The safety, tolerability, and pharmacokinetics of this compound have been evaluated, and interim efficacy and safety results, particularly those from a clinical phase 2a early bactericidal activity trial including patients with drug-susceptible tuberculosis, were reported .
Dosage Effects in Animal Models
In animal studies of systemic infection, soft tissue infection, lung infection, and thigh infection models in mice, this compound showed greater efficacy than Linezolid . The safety, tolerability, and pharmacokinetics of this compound have been evaluated in a phase 1 clinical trial .
Preparation Methods
Delpazolid is synthesized through a series of chemical reactions involving the formation of the oxazolidinone ring and the incorporation of the cyclic amidrazone moiety . The synthetic route typically involves the following steps:
- Formation of the oxazolidinone core through cyclization reactions.
- Introduction of the cyclic amidrazone group via nucleophilic substitution reactions.
- Purification and crystallization to obtain the final product .
Industrial production methods for this compound involve optimizing these synthetic routes to ensure high yield and purity. This includes controlling reaction conditions such as temperature, pressure, and pH, as well as using advanced purification techniques like chromatography .
Chemical Reactions Analysis
Delpazolid undergoes various chemical reactions, including:
Oxidation: this compound can be oxidized under specific conditions to form oxidized derivatives.
Reduction: It can be reduced to form reduced derivatives, which may have different biological activities.
Substitution: This compound can undergo nucleophilic substitution reactions, particularly at the cyclic amidrazone moiety.
Common reagents used in these reactions include oxidizing agents like hydrogen peroxide, reducing agents like sodium borohydride, and nucleophiles like amines . The major products formed from these reactions are typically derivatives of this compound with modified biological activities .
Scientific Research Applications
Delpazolid has a wide range of scientific research applications, including:
Comparison with Similar Compounds
Delpazolid is compared with other oxazolidinones such as linezolid, sutezolid, and tedizolid . While all these compounds share a similar mechanism of action, this compound has several unique features:
Reduced Myelosuppression: This compound has been shown to cause less myelosuppression compared to linezolid, making it a safer option for long-term use.
Enhanced Activity: This compound exhibits greater inhibitory effects against certain bacterial strains, including multidrug-resistant Mycobacterium tuberculosis.
Improved Pharmacokinetics: This compound has a better pharmacokinetic profile, with higher bioavailability and lower protein binding.
Similar compounds include:
Properties
IUPAC Name |
(5R)-3-[3-fluoro-4-(1-methyl-5,6-dihydro-1,2,4-triazin-4-yl)phenyl]-5-(hydroxymethyl)-1,3-oxazolidin-2-one | |
---|---|---|
Source | PubChem | |
URL | https://pubchem.ncbi.nlm.nih.gov | |
Description | Data deposited in or computed by PubChem | |
InChI |
InChI=1S/C14H17FN4O3/c1-17-4-5-18(9-16-17)13-3-2-10(6-12(13)15)19-7-11(8-20)22-14(19)21/h2-3,6,9,11,20H,4-5,7-8H2,1H3/t11-/m1/s1 | |
Source | PubChem | |
URL | https://pubchem.ncbi.nlm.nih.gov | |
Description | Data deposited in or computed by PubChem | |
InChI Key |
QLUWQAFDTNAYPN-LLVKDONJSA-N | |
Source | PubChem | |
URL | https://pubchem.ncbi.nlm.nih.gov | |
Description | Data deposited in or computed by PubChem | |
Canonical SMILES |
CN1CCN(C=N1)C2=C(C=C(C=C2)N3CC(OC3=O)CO)F | |
Source | PubChem | |
URL | https://pubchem.ncbi.nlm.nih.gov | |
Description | Data deposited in or computed by PubChem | |
Isomeric SMILES |
CN1CCN(C=N1)C2=C(C=C(C=C2)N3C[C@@H](OC3=O)CO)F | |
Source | PubChem | |
URL | https://pubchem.ncbi.nlm.nih.gov | |
Description | Data deposited in or computed by PubChem | |
Molecular Formula |
C14H17FN4O3 | |
Source | PubChem | |
URL | https://pubchem.ncbi.nlm.nih.gov | |
Description | Data deposited in or computed by PubChem | |
Molecular Weight |
308.31 g/mol | |
Source | PubChem | |
URL | https://pubchem.ncbi.nlm.nih.gov | |
Description | Data deposited in or computed by PubChem | |
CAS No. |
1219707-39-7 | |
Record name | LCB01-0371 | |
Source | ChemIDplus | |
URL | https://pubchem.ncbi.nlm.nih.gov/substance/?source=chemidplus&sourceid=1219707397 | |
Description | ChemIDplus is a free, web search system that provides access to the structure and nomenclature authority files used for the identification of chemical substances cited in National Library of Medicine (NLM) databases, including the TOXNET system. | |
Record name | LCB01-0371 | |
Source | DrugBank | |
URL | https://www.drugbank.ca/drugs/DB13077 | |
Description | The DrugBank database is a unique bioinformatics and cheminformatics resource that combines detailed drug (i.e. chemical, pharmacological and pharmaceutical) data with comprehensive drug target (i.e. sequence, structure, and pathway) information. | |
Explanation | Creative Common's Attribution-NonCommercial 4.0 International License (http://creativecommons.org/licenses/by-nc/4.0/legalcode) | |
Record name | DELPAZOLID | |
Source | FDA Global Substance Registration System (GSRS) | |
URL | https://gsrs.ncats.nih.gov/ginas/app/beta/substances/43EP6XV33E | |
Description | The FDA Global Substance Registration System (GSRS) enables the efficient and accurate exchange of information on what substances are in regulated products. Instead of relying on names, which vary across regulatory domains, countries, and regions, the GSRS knowledge base makes it possible for substances to be defined by standardized, scientific descriptions. | |
Explanation | Unless otherwise noted, the contents of the FDA website (www.fda.gov), both text and graphics, are not copyrighted. They are in the public domain and may be republished, reprinted and otherwise used freely by anyone without the need to obtain permission from FDA. Credit to the U.S. Food and Drug Administration as the source is appreciated but not required. | |
Disclaimer and Information on In-Vitro Research Products
Please be aware that all articles and product information presented on BenchChem are intended solely for informational purposes. The products available for purchase on BenchChem are specifically designed for in-vitro studies, which are conducted outside of living organisms. In-vitro studies, derived from the Latin term "in glass," involve experiments performed in controlled laboratory settings using cells or tissues. It is important to note that these products are not categorized as medicines or drugs, and they have not received approval from the FDA for the prevention, treatment, or cure of any medical condition, ailment, or disease. We must emphasize that any form of bodily introduction of these products into humans or animals is strictly prohibited by law. It is essential to adhere to these guidelines to ensure compliance with legal and ethical standards in research and experimentation.