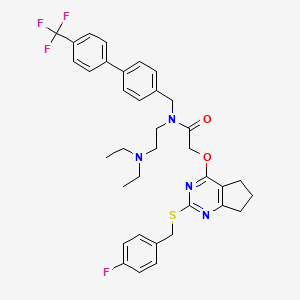
Darapladib-impurity
Overview
Description
Darapladib-impurity is a chemical compound that is an impurity of Darapladib, a selective inhibitor of lipoprotein-associated phospholipase A2 (Lp-PLA2). Darapladib is primarily investigated for its potential in treating atherosclerosis by inhibiting the enzyme Lp-PLA2, which is involved in the inflammatory processes associated with cardiovascular diseases .
Mechanism of Action
- Darapladib is a selective inhibitor of lipoprotein-associated phospholipase A2 (Lp-PLA2) . Lp-PLA2 plays a crucial role in inflammatory processes.
- Ferroptosis is a form of programmed necrosis that requires free active iron and involves lipid peroxidation .
- Lipidomic analyses reveal that Darapladib treatment enriches phosphatidylethanolamine species and reduces lysophosphatidylethanolamine species .
- Darapladib effectively inhibits plasma Lp-PLA2 activity both ex vivo (in human plasma) and in vivo (in Watanabe hereditable hyperlipidaemic rabbit plasma) .
Target of Action
Mode of Action
Biochemical Pathways
Pharmacokinetics
Result of Action
Biochemical Analysis
Biochemical Properties
Darapladib-Impurity, like Darapladib, is likely to interact with Lp-PLA2, an enzyme that plays a key role in intracellular phospholipid metabolism . The interaction between this compound and Lp-PLA2 could potentially influence the balance of phosphatidylethanolamine species and lysophosphatidylethanolamine species within cells .
Cellular Effects
In the context of cancer cells, this compound may enhance ferroptosis, a form of programmed cell death, under lipoprotein-deficient or serum-free conditions . This suggests that this compound could have a significant impact on cell signaling pathways, gene expression, and cellular metabolism .
Molecular Mechanism
The molecular mechanism of this compound likely involves its inhibition of Lp-PLA2, which in turn affects lipid metabolism within cells . This could lead to changes in gene expression and enzyme activity, potentially influencing the susceptibility of cells to ferroptosis .
Temporal Effects in Laboratory Settings
While specific studies on the temporal effects of this compound in laboratory settings are limited, research on Darapladib suggests that it can have long-term effects on cellular function . For instance, Darapladib has been shown to reduce major coronary events over a median follow-up period of 3.7 years .
Dosage Effects in Animal Models
Studies on Darapladib have shown that it can inhibit tumor growth in a xenograft model when combined with the GPX4 inhibitor PACMA31 .
Metabolic Pathways
This compound is likely to be involved in the metabolic pathway of phospholipids, given its interaction with Lp-PLA2 . This could potentially affect metabolic flux or metabolite levels within cells .
Transport and Distribution
Darapladib is known to be lipophilic with good cell membrane permeability , which could also apply to this compound.
Subcellular Localization
Lp-PLA2, the enzyme that this compound likely interacts with, is known to be located in the membrane and cytoplasm . This suggests that this compound could also be found in these subcellular locations.
Preparation Methods
The synthesis of Darapladib-impurity involves several steps, including the formation of key intermediates and their subsequent reactions. The synthetic route typically includes the use of various reagents and catalysts under controlled conditions. Industrial production methods for this compound are not extensively documented, but they generally follow similar principles as those used in laboratory synthesis, with adjustments for scale and efficiency .
Chemical Reactions Analysis
Darapladib-impurity undergoes several types of chemical reactions, including:
Oxidation: This reaction involves the addition of oxygen or the removal of hydrogen. Common reagents include oxidizing agents like potassium permanganate or hydrogen peroxide.
Reduction: This reaction involves the addition of hydrogen or the removal of oxygen. Common reagents include reducing agents like lithium aluminum hydride or sodium borohydride.
Substitution: This reaction involves the replacement of one atom or group of atoms with another. Common reagents include halogens and nucleophiles.
The major products formed from these reactions depend on the specific conditions and reagents used .
Scientific Research Applications
Darapladib-impurity is primarily used in scientific research to study the effects of impurities on the efficacy and safety of Darapladib. It is also used to investigate the metabolic pathways and interactions of Darapladib in biological systems. In addition, this compound has applications in the development of analytical methods for detecting and quantifying impurities in pharmaceutical formulations .
Comparison with Similar Compounds
Darapladib-impurity is unique in its specific inhibition of Lp-PLA2. Similar compounds include other phospholipase A2 inhibitors, such as:
Methyl arachidonyl fluorophosphonate: A potent inhibitor of cytosolic phospholipase A2.
Bromoenol lactone: An inhibitor of calcium-independent phospholipase A2.
Arachidonyl trifluoromethyl ketone: An inhibitor of both cytosolic and calcium-independent phospholipase A2.
Compared to these compounds, this compound is more selective for lipoprotein-associated phospholipase A2, making it particularly useful for studying the specific role of this enzyme in inflammatory processes .
Biological Activity
Darapladib is a potent inhibitor of lipoprotein-associated phospholipase A2 (Lp-PLA2), an enzyme implicated in the pathophysiology of cardiovascular diseases. This article will explore the biological activity of darapladib, including its mechanism of action, effects on cardiovascular biomarkers, and relevant case studies.
Darapladib functions primarily as a reversible inhibitor of Lp-PLA2. This enzyme hydrolyzes oxidized phospholipids, which are known to contribute to inflammation and atherosclerosis. By inhibiting Lp-PLA2, darapladib reduces the formation of pro-inflammatory mediators such as lysophosphatidylcholine and oxidized free fatty acids, potentially stabilizing atherosclerotic plaques and reducing cardiovascular risk .
2.1 Inhibition of Lp-PLA2
Darapladib has demonstrated significant inhibitory effects on Lp-PLA2 activity across various studies:
- IC50 Values : The compound exhibits an IC50 of approximately 0.25 nM against human recombinant Lp-PLA2 in vitro . In whole human plasma, this value is reported to be around 5 nM .
- Dose-Dependent Effects : Clinical trials have shown that darapladib can achieve a reduction in Lp-PLA2 levels by 60-66% at a daily dose of 160 mg .
2.2 Impact on Inflammatory Biomarkers
In addition to inhibiting Lp-PLA2, darapladib has been associated with changes in various inflammatory biomarkers:
- Interleukin-6 (IL-6) : A modest reduction of 12.3% was observed after treatment with darapladib compared to placebo .
- High-Sensitivity C-Reactive Protein (hs-CRP) : There was a non-significant reduction of 13% in hs-CRP levels .
3.1 STABILITY Trial
The STABILITY trial investigated the efficacy of darapladib in patients with stable coronary artery disease (CAD):
- Study Design : A total of 15,828 patients were randomly assigned to receive either darapladib (160 mg daily) or placebo over a median follow-up period of 3.7 years.
- Outcomes : The primary endpoint, which included major adverse cardiovascular events (MACE), was not significantly reduced (hazard ratio 0.94; p=0.20). However, there was a significant reduction in non-fatal myocardial infarctions (HR 0.90; p=0.045) and total coronary events (HR 0.91; p=0.02) .
3.2 Other Observations
In preclinical studies involving swine models, darapladib showed promising results in reducing inflammatory effects and stabilizing atherosclerotic plaques by halting necrotic core formation .
4. Safety Profile
The safety profile of darapladib has generally been consistent across studies, with no major safety concerns reported. However, some patients experienced gastrointestinal side effects leading to discontinuation .
5. Summary Table of Key Findings
Study/Trial | Population Size | Primary Endpoint | Key Findings |
---|---|---|---|
STABILITY Trial | 15,828 | MACE | No significant reduction; HR 0.94 (p=0.20) |
Inflammatory Markers | Various | IL-6 & hs-CRP | IL-6 reduced by 12.3%; hs-CRP reduced by 13% |
Preclinical Swine Study | N/A | Atherosclerosis | Reduced necrotic core formation observed |
6. Conclusion
Darapladib presents a unique approach to managing cardiovascular disease through the inhibition of Lp-PLA2 and modulation of inflammatory pathways. While it has shown promise in reducing specific cardiovascular risk markers and stabilizing plaque composition, its overall impact on major cardiovascular events remains inconclusive based on current clinical trials.
Future research should focus on long-term outcomes and potential combinations with other therapeutic agents to enhance efficacy while monitoring safety profiles closely.
Properties
IUPAC Name |
N-[2-(diethylamino)ethyl]-2-[[2-[(4-fluorophenyl)methylsulfanyl]-6,7-dihydro-5H-cyclopenta[d]pyrimidin-4-yl]oxy]-N-[[4-[4-(trifluoromethyl)phenyl]phenyl]methyl]acetamide | |
---|---|---|
Source | PubChem | |
URL | https://pubchem.ncbi.nlm.nih.gov | |
Description | Data deposited in or computed by PubChem | |
InChI |
InChI=1S/C36H38F4N4O2S/c1-3-43(4-2)20-21-44(22-25-8-12-27(13-9-25)28-14-16-29(17-15-28)36(38,39)40)33(45)23-46-34-31-6-5-7-32(31)41-35(42-34)47-24-26-10-18-30(37)19-11-26/h8-19H,3-7,20-24H2,1-2H3 | |
Source | PubChem | |
URL | https://pubchem.ncbi.nlm.nih.gov | |
Description | Data deposited in or computed by PubChem | |
InChI Key |
KELIVYXNVITYCV-UHFFFAOYSA-N | |
Source | PubChem | |
URL | https://pubchem.ncbi.nlm.nih.gov | |
Description | Data deposited in or computed by PubChem | |
Canonical SMILES |
CCN(CC)CCN(CC1=CC=C(C=C1)C2=CC=C(C=C2)C(F)(F)F)C(=O)COC3=NC(=NC4=C3CCC4)SCC5=CC=C(C=C5)F | |
Source | PubChem | |
URL | https://pubchem.ncbi.nlm.nih.gov | |
Description | Data deposited in or computed by PubChem | |
Molecular Formula |
C36H38F4N4O2S | |
Source | PubChem | |
URL | https://pubchem.ncbi.nlm.nih.gov | |
Description | Data deposited in or computed by PubChem | |
Molecular Weight |
666.8 g/mol | |
Source | PubChem | |
URL | https://pubchem.ncbi.nlm.nih.gov | |
Description | Data deposited in or computed by PubChem | |
Retrosynthesis Analysis
AI-Powered Synthesis Planning: Our tool employs the Template_relevance Pistachio, Template_relevance Bkms_metabolic, Template_relevance Pistachio_ringbreaker, Template_relevance Reaxys, Template_relevance Reaxys_biocatalysis model, leveraging a vast database of chemical reactions to predict feasible synthetic routes.
One-Step Synthesis Focus: Specifically designed for one-step synthesis, it provides concise and direct routes for your target compounds, streamlining the synthesis process.
Accurate Predictions: Utilizing the extensive PISTACHIO, BKMS_METABOLIC, PISTACHIO_RINGBREAKER, REAXYS, REAXYS_BIOCATALYSIS database, our tool offers high-accuracy predictions, reflecting the latest in chemical research and data.
Strategy Settings
Precursor scoring | Relevance Heuristic |
---|---|
Min. plausibility | 0.01 |
Model | Template_relevance |
Template Set | Pistachio/Bkms_metabolic/Pistachio_ringbreaker/Reaxys/Reaxys_biocatalysis |
Top-N result to add to graph | 6 |
Feasible Synthetic Routes
Disclaimer and Information on In-Vitro Research Products
Please be aware that all articles and product information presented on BenchChem are intended solely for informational purposes. The products available for purchase on BenchChem are specifically designed for in-vitro studies, which are conducted outside of living organisms. In-vitro studies, derived from the Latin term "in glass," involve experiments performed in controlled laboratory settings using cells or tissues. It is important to note that these products are not categorized as medicines or drugs, and they have not received approval from the FDA for the prevention, treatment, or cure of any medical condition, ailment, or disease. We must emphasize that any form of bodily introduction of these products into humans or animals is strictly prohibited by law. It is essential to adhere to these guidelines to ensure compliance with legal and ethical standards in research and experimentation.