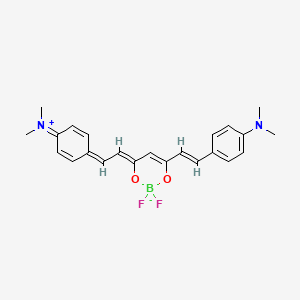
Cranad-2
- Click on QUICK INQUIRY to receive a quote from our team of experts.
- With the quality product at a COMPETITIVE price, you can focus more on your research.
Overview
Description
Cranad-2 is a curcumin derivative that has gained significant attention in scientific research due to its unique properties. It is primarily used as a near-infrared fluorescent probe for detecting amyloid-beta deposits, which are associated with Alzheimer’s disease . This compound exhibits excellent optical characteristics, making it a valuable tool for in vivo imaging and diagnostic applications .
Scientific Research Applications
Cranad-2 has a wide range of scientific research applications, including:
Mechanism of Action
Target of Action
Cranad-2 is a near-infrared (NIR) fluorescent probe that primarily targets Amyloid-β (Aβ) plaques . These plaques are protein aggregates that are a major histopathological hallmark of Alzheimer’s disease . This compound has a high affinity for Aβ aggregates, with a dissociation constant (Kd) of 38 nM .
Mode of Action
Upon interacting with Aβ aggregates, this compound exhibits a significant increase in fluorescence intensity . This interaction results in a 70-fold increase in fluorescence intensity and a 90 nm blue shift from 805 to 715 nm . This change in fluorescence properties allows for the detection and imaging of Aβ plaques .
Biochemical Pathways
It is known that the probe’s interaction with aβ plaques can help visualize these structures in the brain, which are associated with the pathogenesis of alzheimer’s disease . The ability to detect these plaques can provide valuable insights into the progression of the disease and the effectiveness of potential treatments.
Pharmacokinetics
This compound is capable of penetrating the blood-brain barrier, which is crucial for its role in detecting Aβ plaques in the brain . This property enhances its bioavailability in the brain, allowing it to interact with its target structures.
Result of Action
The primary result of this compound’s action is the enhanced visualization of Aβ plaques. Its interaction with these plaques leads to a significant increase in fluorescence intensity, which can be detected using appropriate imaging techniques . This allows for the in vivo detection of Aβ plaques, which can be crucial in the study and diagnosis of Alzheimer’s disease .
Action Environment
The action of this compound is influenced by its ability to penetrate the blood-brain barrier, which allows it to reach its target Aβ plaques in the brain
Safety and Hazards
Future Directions
Cranad-2 has been used for multi-spectral optoacoustic tomography and fluorescence imaging of brain Aβ deposits in the arcAβ mouse model of AD . It has shown potential for use in fluorescence- and MSOT-based detection of Aβ deposits in animal models of AD pathology . This facilitates mechanistic studies and the monitoring of putative treatments targeting Aβ deposits .
Biochemical Analysis
Biochemical Properties
Cranad-2 has a high affinity for amyloid-β aggregates, with a dissociation constant (Kd) of 38 nM . Upon interacting with Amyloid-β aggregates, it displays a 70-fold increase in fluorescence intensity accompanied by a 90 nm blue shift from 805 to 715 nm .
Cellular Effects
This compound has been shown to detect amyloid-β in vitro and in vivo . It is capable of distinguishing between monomeric and fibrillar forms of Aβ . This suggests that this compound may influence cell function by interacting with amyloid-β aggregates, potentially impacting cell signaling pathways, gene expression, and cellular metabolism.
Molecular Mechanism
The molecular mechanism of this compound involves its interaction with amyloid-β aggregates. The binding of this compound to these aggregates results in a significant increase in fluorescence intensity and a shift in the emission wavelength . This suggests that this compound may exert its effects at the molecular level through binding interactions with amyloid-β aggregates.
Temporal Effects in Laboratory Settings
In laboratory settings, this compound has been used for the detection of Aβ deposits in the arcAβ mouse model of Alzheimer’s disease cerebral amyloidosis
Transport and Distribution
This compound is known to be blood-brain barrier permeable , suggesting that it can be transported and distributed within cells and tissues
Preparation Methods
Synthetic Routes and Reaction Conditions
Cranad-2 is synthesized through a multi-step chemical process involving the modification of curcumin. The reaction conditions often include the use of organic solvents and catalysts to facilitate the desired chemical transformations .
Industrial Production Methods
While specific industrial production methods for this compound are not extensively documented, the synthesis process can be scaled up using standard organic synthesis techniques. The key steps involve the preparation of intermediate compounds, followed by their purification and final modification to obtain this compound with high purity .
Chemical Reactions Analysis
Types of Reactions
Cranad-2 undergoes various chemical reactions, including:
Oxidation: This compound can be oxidized under specific conditions, leading to changes in its optical properties.
Common Reagents and Conditions
Oxidation: Common oxidizing agents include hydrogen peroxide and potassium permanganate.
Reduction: Reducing agents such as sodium borohydride and lithium aluminum hydride are often used.
Substitution: Various nucleophiles and electrophiles can be employed in substitution reactions, depending on the desired modification.
Major Products Formed
The major products formed from these reactions depend on the specific reagents and conditions used. For example, oxidation may result in the formation of oxidized derivatives with altered fluorescence properties .
Comparison with Similar Compounds
Cranad-2 is compared with other curcumin-based fluorescent probes, such as Cranad-5 and Cranad-61 . While all these compounds share a similar curcumin scaffold, this compound is unique due to its specific optical properties and high binding affinity for amyloid-beta aggregates . The table below highlights the key differences:
Compound | Unique Features | Binding Affinity (Kd) |
---|---|---|
This compound | Near-infrared fluorescence, high sensitivity | 38 nM |
Cranad-5 | Suitable for FRET applications | 10 nM |
Cranad-61 | Emission wavelength difference due to oxalate moiety | Not specified |
Similar Compounds
- Cranad-5
- Cranad-61
- Bisdemethoxycurcumin
This compound stands out due to its excellent optical characteristics and high specificity for amyloid-beta aggregates, making it a valuable tool in Alzheimer’s disease research and diagnostics .
Properties
{ "Design of the Synthesis Pathway": "The synthesis pathway of Cranad-2 involves the reaction of two starting materials: 4,7-dibromo-2,1,3-benzothiadiazole and 4-(diphenylamino)phenyl)boronic acid. The reaction is carried out using a palladium-catalyzed Suzuki-Miyaura cross-coupling reaction.", "Starting Materials": [ "4,7-dibromo-2,1,3-benzothiadiazole", "4-(diphenylamino)phenyl)boronic acid" ], "Reaction": [ "Step 1: 4,7-dibromo-2,1,3-benzothiadiazole is dissolved in anhydrous dimethylformamide (DMF) and heated to 80-90°C under nitrogen atmosphere.", "Step 2: To the above solution, 4-(diphenylamino)phenyl)boronic acid, palladium acetate, and potassium carbonate are added sequentially.", "Step 3: The reaction mixture is stirred at 80-90°C for 12-24 hours.", "Step 4: After completion of the reaction, the mixture is cooled to room temperature and poured into water.", "Step 5: The resulting precipitate is filtered and washed with water and methanol.", "Step 6: The crude product is purified by column chromatography using a mixture of dichloromethane and methanol as the eluent.", "Step 7: The purified product is obtained as a yellow powder and characterized by various spectroscopic techniques such as nuclear magnetic resonance (NMR) and mass spectrometry (MS)." ] } | |
CAS No. |
1193447-34-5 |
Molecular Formula |
C23H25BF2N2O2 |
Molecular Weight |
410.3 g/mol |
IUPAC Name |
[4-[2-[6-[(E)-2-[4-(dimethylamino)phenyl]ethenyl]-2,2-difluoro-1,3-dioxa-2-boranuidacyclohex-5-en-4-ylidene]ethylidene]cyclohexa-2,5-dien-1-ylidene]-dimethylazanium |
InChI |
InChI=1S/C23H25BF2N2O2/c1-27(2)20-11-5-18(6-12-20)9-15-22-17-23(30-24(25,26)29-22)16-10-19-7-13-21(14-8-19)28(3)4/h5-17H,1-4H3 |
InChI Key |
PVXQJYXODFZTBR-UHFFFAOYSA-N |
Isomeric SMILES |
[B-]1(OC(=CC(=CC=C2C=CC(=[N+](C)C)C=C2)O1)/C=C/C3=CC=C(C=C3)N(C)C)(F)F |
SMILES |
[B-]1(OC(=CC(=CC=C2C=CC(=[N+](C)C)C=C2)O1)C=CC3=CC=C(C=C3)N(C)C)(F)F |
Canonical SMILES |
[B-]1(OC(=CC(=CC=C2C=CC(=[N+](C)C)C=C2)O1)C=CC3=CC=C(C=C3)N(C)C)(F)F |
Appearance |
Solid powder |
Purity |
>98% (or refer to the Certificate of Analysis) |
shelf_life |
>2 years if stored properly |
solubility |
Soluble in DMSO, not in water |
storage |
Dry, dark and at 0 - 4 C for short term (days to weeks) or -20 C for long term (months to years). |
Synonyms |
CRANAD-2; CRANAD 2; CRANAD2. |
Origin of Product |
United States |
Disclaimer and Information on In-Vitro Research Products
Please be aware that all articles and product information presented on BenchChem are intended solely for informational purposes. The products available for purchase on BenchChem are specifically designed for in-vitro studies, which are conducted outside of living organisms. In-vitro studies, derived from the Latin term "in glass," involve experiments performed in controlled laboratory settings using cells or tissues. It is important to note that these products are not categorized as medicines or drugs, and they have not received approval from the FDA for the prevention, treatment, or cure of any medical condition, ailment, or disease. We must emphasize that any form of bodily introduction of these products into humans or animals is strictly prohibited by law. It is essential to adhere to these guidelines to ensure compliance with legal and ethical standards in research and experimentation.