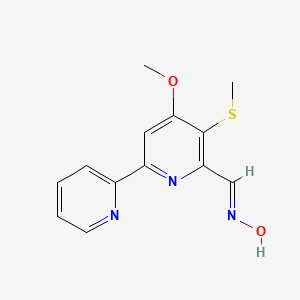
Collismycin A
Overview
Description
Collismycin A is a natural product belonging to the 2,2’-bipyridyl family of compounds. It is produced by the bacterium Streptomyces globisporus and has been found to exhibit significant biological activities, including cytotoxic and antimicrobial properties . This compound is particularly noted for its potential as an iron chelator, which has implications for its use in various therapeutic applications .
Mechanism of Action
Target of Action
Collismycin A, a microbial product, primarily targets iron ions in the body . Iron plays a crucial role in various biological processes, including oxygen transport, DNA synthesis, and electron transport . This compound binds to both Fe(II) and Fe(III) ions, forming a 2:1 chelator-iron complex with a redox-inactive center .
Mode of Action
This compound acts as a specific iron chelator . It binds to iron ions, forming a complex that inhibits the normal function of iron in the body .
Biochemical Pathways
The primary biochemical pathway affected by this compound is the glycolytic pathway . The compound’s interaction with iron ions leads to the accumulation of HIF-1α, a transcription factor that plays a key role in the regulation of genes involved in energy metabolism, angiogenesis, and apoptosis . This accumulation affects the glycolytic pathway, potentially leading to changes in cellular metabolism .
Pharmacokinetics
The compound’s ability to form a complex with iron ions suggests that it may have a high affinity for these ions, which could influence its bioavailability .
Result of Action
The primary result of this compound’s action is the inhibition of cancer cell growth . By chelating iron ions, the compound disrupts normal cellular processes, leading to cell growth inhibition . In addition, the compound’s effect on the glycolytic pathway may lead to changes in cellular metabolism, further contributing to its anti-proliferative activity .
Biochemical Analysis
Biochemical Properties
Collismycin A interacts with both Fe(II) and Fe(III) ions, forming a 2:1 chelator-iron complex with a redox-inactive center . This interaction plays a significant role in its biochemical reactions. The compound’s anti-proliferative activity against cancer cells is completely canceled by Fe(II) and Fe(III) ions, but not by other metal ions such as Zn(II) or Cu(II) .
Cellular Effects
This compound has been found to have a significant impact on various types of cells and cellular processes. It inhibits the proliferation of A549 lung, HCT116 colon, and HeLa cervical cancer cells . It does not inhibit the proliferation of MDA-MB-231 breast cancer cells . The compound also affects the glycolytic pathway due to the accumulation of HIF-1α .
Molecular Mechanism
The molecular mechanism of this compound involves its action as a specific iron chelator . By binding to both Fe(II) and Fe(III) ions, it forms a 2:1 chelator-iron complex with a redox-inactive center . This binding interaction leads to the inhibition of cancer cell growth .
Temporal Effects in Laboratory Settings
It is known that the compound’s anti-proliferative activity against cancer cells can be completely canceled by Fe(II) and Fe(III) ions .
Metabolic Pathways
This compound is involved in the glycolytic pathway due to the accumulation of HIF-1α
Preparation Methods
Synthetic Routes and Reaction Conditions
Collismycin A is typically isolated from the fermentation broth of Streptomyces globisporus. The production involves cultivating the bacterium under specific conditions that promote the biosynthesis of this compound. The isolation process includes extraction with organic solvents followed by purification using chromatographic techniques .
Industrial Production Methods
While the industrial production of this compound is not extensively documented, it generally follows the principles of microbial fermentation. Large-scale fermentation processes are optimized to maximize yield, and downstream processing involves extraction and purification to obtain the pure compound .
Chemical Reactions Analysis
Types of Reactions
Collismycin A undergoes several types of chemical reactions, including:
Oxidation: This reaction can alter the functional groups present in the molecule, potentially modifying its biological activity.
Reduction: Reduction reactions can be used to modify the oxidation state of the compound, affecting its reactivity and stability.
Common Reagents and Conditions
Oxidation: Common oxidizing agents include hydrogen peroxide and potassium permanganate.
Reduction: Reducing agents such as sodium borohydride and lithium aluminum hydride are often used.
Substitution: Reagents like halogens and nucleophiles are employed under specific conditions to achieve substitution reactions.
Major Products Formed
The major products formed from these reactions depend on the specific reagents and conditions used. For example, oxidation may yield various oxidized derivatives, while substitution reactions can produce a range of functionalized analogs .
Scientific Research Applications
Collismycin A has a wide range of scientific research applications:
Medicine: this compound’s cytotoxic properties make it a candidate for anticancer research.
Industry: The compound’s antimicrobial properties are explored for potential use in developing new antibiotics.
Comparison with Similar Compounds
Similar Compounds
Caerulomycin A: Another member of the 2,2’-bipyridyl family, known for its immunosuppressive and antimicrobial properties.
Actinomycin X2: A compound with similar antimicrobial activity, produced by the same bacterium, Streptomyces globisporus.
Uniqueness
Collismycin A is unique due to its dual role as an iron chelator and a cytotoxic agent. This combination of properties makes it particularly valuable for research into treatments for cancer and microbial infections. Its ability to form stable complexes with iron ions distinguishes it from other similar compounds .
Properties
IUPAC Name |
(NE)-N-[(4-methoxy-3-methylsulfanyl-6-pyridin-2-ylpyridin-2-yl)methylidene]hydroxylamine | |
---|---|---|
Source | PubChem | |
URL | https://pubchem.ncbi.nlm.nih.gov | |
Description | Data deposited in or computed by PubChem | |
InChI |
InChI=1S/C13H13N3O2S/c1-18-12-7-10(9-5-3-4-6-14-9)16-11(8-15-17)13(12)19-2/h3-8,17H,1-2H3/b15-8+ | |
Source | PubChem | |
URL | https://pubchem.ncbi.nlm.nih.gov | |
Description | Data deposited in or computed by PubChem | |
InChI Key |
NQGMIPUYCWIEAW-OVCLIPMQSA-N | |
Source | PubChem | |
URL | https://pubchem.ncbi.nlm.nih.gov | |
Description | Data deposited in or computed by PubChem | |
Canonical SMILES |
COC1=CC(=NC(=C1SC)C=NO)C2=CC=CC=N2 | |
Source | PubChem | |
URL | https://pubchem.ncbi.nlm.nih.gov | |
Description | Data deposited in or computed by PubChem | |
Isomeric SMILES |
COC1=CC(=NC(=C1SC)/C=N/O)C2=CC=CC=N2 | |
Source | PubChem | |
URL | https://pubchem.ncbi.nlm.nih.gov | |
Description | Data deposited in or computed by PubChem | |
Molecular Formula |
C13H13N3O2S | |
Source | PubChem | |
URL | https://pubchem.ncbi.nlm.nih.gov | |
Description | Data deposited in or computed by PubChem | |
Molecular Weight |
275.33 g/mol | |
Source | PubChem | |
URL | https://pubchem.ncbi.nlm.nih.gov | |
Description | Data deposited in or computed by PubChem | |
CAS No. |
158792-24-6 | |
Record name | Collismycin A | |
Source | ChemIDplus | |
URL | https://pubchem.ncbi.nlm.nih.gov/substance/?source=chemidplus&sourceid=0158792246 | |
Description | ChemIDplus is a free, web search system that provides access to the structure and nomenclature authority files used for the identification of chemical substances cited in National Library of Medicine (NLM) databases, including the TOXNET system. | |
Q1: What is the primary mechanism of action of Collismycin A?
A1: this compound acts as an iron chelator. [, ] This means it binds to iron ions, making them unavailable for cellular processes.
Q2: How does iron chelation by this compound affect tumor cells?
A2: Iron is crucial for tumor cell growth and proliferation. By chelating iron, this compound inhibits the growth of various human tumor cells, with IC50 values ranging from 100 to 400 nM. []
Q3: What specific cellular processes are affected by this compound's iron chelation activity?
A3: this compound treatment leads to cell cycle arrest at the G1 phase and a marked decrease in Cyclin D1 levels. [] This suggests its iron chelation disrupts cell cycle progression.
Q4: Does this compound show selectivity for iron over other metal ions?
A4: Yes, this compound demonstrates a preference for iron. Its antiproliferative activity is completely abolished by excess iron (Fe(II) or Fe(III)) but not by other metal ions like copper (Cu(II)), zinc (Zn(II)), manganese (Mn(II)), or magnesium (Mg(II)). []
Q5: How does this compound's mechanism compare to other antifungal agents?
A5: Unlike some antifungals that target specific fungal proteins, this compound disrupts iron homeostasis, a process essential for fungal survival, particularly during infection. [] This makes it effective against various Candida species, including drug-resistant strains.
Q6: Does Collismycin C, another compound in the collismycin family, share a similar mechanism of action?
A6: While both compounds possess a 2,2′-bipyridine structure, Collismycin C primarily targets the High Mobility Group Box 1 (HMGB1) protein, a crucial mediator in septic responses. [] It inhibits HMGB1 release and downstream inflammatory responses.
Q7: What is the molecular formula and weight of this compound?
A7: The molecular formula of this compound is C22H21N3O6S, and its molecular weight is 455.50 g/mol. [, ]
Q8: What spectroscopic techniques are commonly employed to characterize this compound?
A8: Nuclear Magnetic Resonance (NMR) spectroscopy, including 1H and 13C NMR, along with Mass Spectrometry (MS), are frequently used to elucidate the structure of this compound. [, ]
Q9: What type of biosynthetic pathway is responsible for this compound production?
A10: this compound is produced through a hybrid polyketide synthase/nonribosomal peptide synthetase (PKS/NRPS) pathway. [, ] This complex assembly line incorporates both polyketide and nonribosomal peptide elements.
Q10: What is the role of the flavin-dependent dehydrogenase ColB1 in this compound biosynthesis?
A11: ColB1, recruited by the NRPS module, catalyzes a crucial step: the dehydrogenation of cysteine bound to the peptidyl carrier protein (PCP). [] This modification is essential for the formation of the 2,2'-bipyridine ring.
Q11: How is this compound biosynthesis regulated within the producing organism?
A12: Two pathway-specific regulators, ClmR1 and ClmR2, control this compound production in Streptomyces sp. CS40. [] ClmR1 acts as a repressor, while ClmR2 is an activator.
Q12: Does iron availability affect this compound biosynthesis?
A13: Yes, high iron concentrations inhibit this compound production by repressing the transcription of clmR2, the activator gene. [] This suggests iron-dependent regulation of the biosynthetic pathway.
Q13: How does the presence and position of a hydroxyl group on the 2,2′-bipyridine scaffold influence the antibiofilm activity?
A14: Research indicates that both the presence and the specific position of the hydroxyl group on the 2,2′-bipyridine ring system significantly influence the antibiofilm activity of Collismycin derivatives. []
Q14: Can the biological activity of this compound be modified through structural alterations?
A15: Yes, modifications to the this compound structure can alter its biological activity. For instance, generating analogs with neuroprotective activity has been achieved through mutasynthesis, a process involving genetic manipulation of the biosynthetic pathway. [, ]
Q15: What types of in vitro assays are used to assess the biological activity of this compound?
A16: Researchers often use cell-based assays to evaluate the effects of this compound on cell proliferation, cell cycle progression, and specific protein levels. [, ]
Q16: Has this compound demonstrated efficacy in in vivo models of disease?
A17: Yes, this compound has shown promising results in a Candida infection model using Galleria mellonella larvae. [] Treatment with this compound significantly increased the survival rate of infected larvae.
Q17: What about Collismycin C? Has it shown any promising results in in vivo studies?
A18: Collismycin C has demonstrated efficacy in a mouse model of sepsis. Treatment with Collismycin C effectively reduced HMGB1 release, sepsis-related mortality, and lung damage. []
Q18: What are the potential applications of this compound based on its biological activity?
A19: this compound's iron-chelating and antiproliferative properties make it a potential candidate for anticancer therapy. [] Its antifungal activity against Candida species, including drug-resistant strains, also holds promise for treating fungal infections. []
Q19: What are the potential applications of Collismycin C based on its biological activity?
A20: Collismycin C shows promise as a potential therapeutic agent for treating severe vascular inflammatory diseases, particularly sepsis, due to its ability to inhibit the HMGB1 signaling pathway. []
Q20: What are the key challenges and future research directions for this compound?
A21: Further research is needed to optimize its pharmacological properties, such as improving its bioavailability and understanding its long-term safety profile. Exploring its synergy with existing antifungal agents like fluconazole [] is also crucial for combating drug resistance.
Disclaimer and Information on In-Vitro Research Products
Please be aware that all articles and product information presented on BenchChem are intended solely for informational purposes. The products available for purchase on BenchChem are specifically designed for in-vitro studies, which are conducted outside of living organisms. In-vitro studies, derived from the Latin term "in glass," involve experiments performed in controlled laboratory settings using cells or tissues. It is important to note that these products are not categorized as medicines or drugs, and they have not received approval from the FDA for the prevention, treatment, or cure of any medical condition, ailment, or disease. We must emphasize that any form of bodily introduction of these products into humans or animals is strictly prohibited by law. It is essential to adhere to these guidelines to ensure compliance with legal and ethical standards in research and experimentation.