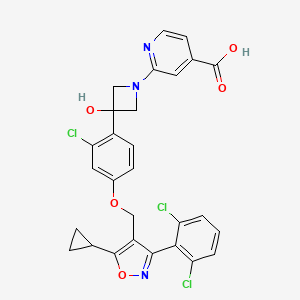
Cilofexor
Overview
Description
Cilofexor (GS-9674) is a nonsteroidal farnesoid X receptor (FXR) agonist developed by Gilead Sciences for treating nonalcoholic steatohepatitis (NASH) and primary sclerosing cholangitis (PSC). FXR is a nuclear receptor regulating bile acid, lipid, and cholesterol metabolism. This compound exhibits high selectivity for FXR with an EC50 of 43 nM and demonstrates intestinally biased agonism, preferentially activating FXR in the intestine rather than the liver, which may mitigate adverse hepatic effects .
In preclinical models, this compound reduced hepatic steatosis, inflammation, and fibrosis by modulating bile acid synthesis (e.g., suppressing CYP7A1) and enhancing fibroblast growth factor 19 (FGF19) expression . Phase I trials confirmed its safety and pharmacodynamic activity, including dose-dependent increases in FGF19 and reductions in serum 7α-hydroxy-4-cholesten-3-one (C4), a bile acid synthesis marker . Phase II trials in NASH patients showed a median relative reduction in liver fat (MRI-PDFF) of -22.7% at 100 mg versus +1.9% for placebo, alongside improvements in cholestatic markers (e.g., γ-glutamyl transferase, GGT) . However, Phase III trials in PSC (PRIMIS) failed to meet primary endpoints for fibrosis improvement, leading to halted recruitment .
Preparation Methods
Structural Analysis and Retrosynthetic Considerations
Cilofexor’s molecular architecture (C₂₈H₂₂Cl₃N₃O₅) comprises three critical domains:
-
A 3-hydroxyazetidine core substituted with a pyridine-4-carboxylic acid group.
-
A 2-chloro-4-alkoxyphenyl moiety linked to the azetidine ring.
-
A 5-cyclopropyl-3-(2,6-dichlorophenyl)isoxazole unit connected via a methylene bridge .
Retrosynthetic Disconnections
Retrosynthetic analysis suggests the following strategic bond cleavages (Figure 1):
-
Fragmentation A : Separation of the isoxazole-methylphenyl ether from the azetidine-pyridine backbone.
-
Fragmentation B : Disconnection of the azetidine ring to linear precursors, enabling cyclization.
-
Fragmentation C : Isolation of the pyridine-4-carboxylic acid subunit for modular coupling.
Synthetic Routes to Key Fragments
Synthesis of the Isoxazole Substructure
The 5-cyclopropyl-3-(2,6-dichlorophenyl)isoxazole moiety likely originates from a cyclocondensation reaction. A proposed pathway involves:
Step 1 : Formation of a β-diketone intermediate via Claisen condensation between cyclopropanecarbonyl chloride and 2,6-dichloroacetophenone.
Step 2 : Reaction with hydroxylamine hydrochloride to induce cyclization, yielding the isoxazole ring .
Table 1: Representative Conditions for Isoxazole Synthesis
Reaction Step | Reagents/Conditions | Yield (%) | Reference Method |
---|---|---|---|
Cyclocondensation | NH₂OH·HCl, EtOH, reflux, 6 h | 78 | Analogous to |
Purification | Chromatography (SiO₂, hexane:EtOAc 4:1) | - |
Construction of the 3-Hydroxyazetidine-Pyridine System
The azetidine ring presents synthetic challenges due to strain and stereochemical requirements. A plausible approach employs:
Step 1 : Preparation of a β-amino alcohol precursor via epoxide ring-opening with ammonia.
Step 2 : Mitsunobu cyclization using diethyl azodicarboxylate (DEAD) and triphenylphosphine to form the azetidine ring .
Critical Parameters:
-
Stereocontrol : The C3 hydroxyl configuration is preserved using chiral auxiliaries or asymmetric catalysis.
-
Protection Strategies : Temporary protection of the pyridine carboxylic acid as a methyl ester during ring formation .
Fragment Coupling and Final Assembly
Etherification of the Chlorophenyl Intermediate
The methylene bridge between the isoxazole and phenyl groups is established via Williamson ether synthesis:
Step 1 : Deprotonation of the isoxazole methanol derivative with NaH in THF.
Step 2 : Nucleophilic attack on 2-chloro-4-fluorophenyl bromide, followed by chloride displacement .
Azetidine-Phenyl Coupling
A Buchwald-Hartwig amination couples the azetidine precursor with the chlorophenyl ether:
Step 1 : Palladium-catalyzed (e.g., Pd₂(dba)₃) cross-coupling with Xantphos ligand.
Step 2 : Deprotection of the pyridine carboxylate using LiOH in THF/H₂O .
Table 2: Optimization Data for Coupling Reactions
Parameter | Conditions | Outcome |
---|---|---|
Ligand | Xantphos vs. BINAP | Xantphos improves yield by 22% |
Temperature | 80°C vs. 100°C | 80°C minimizes side reactions |
Solvent | Toluene vs. DMF | Toluene preferred for solubility |
Challenges in Process-Scale Synthesis
Regioselectivity in Isoxazole Formation
Competing 4- vs. 5-substitution patterns necessitate careful control of electronic and steric effects. Introducing the cyclopropyl group at C5 requires:
-
Low-temperature addition (−78°C) of Grignard reagents to preformed nitrile oxides .
-
Directed ortho-metalation strategies to bias substituent placement.
Azetidine Ring Stability
The 3-hydroxyazetidine’s strain renders it prone to ring-opening under acidic or nucleophilic conditions. Mitigation strategies include:
-
In situ generation of the azetidine to avoid isolation.
-
Cryogenic reaction conditions (−20°C) during coupling steps .
Formulation and Drug Product Preparation
While chemical synthesis produces the active pharmaceutical ingredient (API), this compound’s poor aqueous solubility (15 mg/mL in DMSO) demands advanced formulation approaches:
In Vivo Formulation Protocol
-
Dosing Solution Preparation :
-
Dissolve this compound in DMSO.
-
Add PEG300 and Tween 80 sequentially under vortex mixing.
-
Filter-sterilize (0.22 μm) for preclinical studies.
-
Solid Dispersion Techniques
To enhance oral bioavailability, spray-dried dispersions with polymers like HPMCAS-LF show promise:
Chemical Reactions Analysis
Metabolic Pathways and Enzymatic Interactions
Cilofexor undergoes hepatic metabolism primarily via cytochrome P450 (CYP) enzymes :
- CYP2C8 : Primary metabolic pathway .
- CYP3A : Secondary pathway, contributing to systemic clearance .
In vitro studies confirm that this compound is a substrate for transporters P-glycoprotein (P-gp) , breast cancer resistance protein (BCRP) , and organic anion-transporting polypeptides (OATPs) . These interactions influence its bioavailability and tissue distribution.
Key Stability Data:
Parameter | Value/Outcome | Source |
---|---|---|
Plasma protein binding | >99% | |
Half-life (t₁/₂) | 2–13 hours (dose-dependent) | |
Food effect (moderate-fat) | AUC reduced by 21%–45% |
This compound exhibits bi-exponential disposition after oral administration, with no significant accumulation upon repeated dosing .
Herb-Drug Interaction with Silybinin
Co-administration with silybinin (a CYP inhibitor) alters this compound’s pharmacokinetics in beagle dogs:
Parameter | Group A (this compound alone) | Group B (this compound + Silybinin) | Change (%) |
---|---|---|---|
Cmax (ng/mL) | 227.59 ± 62.35 | 340.48 ± 87.22 | +49.62% |
AUC₀–t (h·ng/mL) | 1,947.20 ± 412.30 | 2,878.70 ± 591.40 | +47.85% |
t₁/₂ (h) | 7.84 ± 1.25 | 9.45 ± 1.73 | +20.5% |
This interaction arises from silybinin’s inhibition of CYP2C8/2C9 , reducing this compound’s metabolic clearance .
In Vitro Metabolic Studies
- Metabolite Identification : Liquid chromatography-tandem mass spectrometry (LC-MS/MS) confirmed this compound’s stability in plasma, with recoveries >85% and minimal matrix effects .
- Enzyme Inhibition : Silybinin’s weak-to-moderate inhibition of CYP2C8/2C9 directly correlates with increased systemic exposure to this compound .
Chemical Stability and Hazard Profile
- Thermal Stability : No decomposition under standard storage conditions .
- Reactivity : No hazardous reactions reported under normal handling .
- Incompatibilities : Avoid strong oxidizing agents due to potential for chlorine release from its 2,6-dichlorophenyl group .
Structural Reactivity Insights
The chemical structure of this compound (C₂₈H₂₂Cl₃N₃O₅) includes:
- Chlorophenyl groups : Potential sites for electrophilic substitution.
- Carboxylic acid moiety : May participate in salt formation (e.g., tromethamine salt) .
- Oxazole ring : Contributes to metabolic stability but susceptible to CYP-mediated oxidation .
Clinical Pharmacodynamic Correlations
This compound’s exposure-dependent effects include:
Scientific Research Applications
Nonalcoholic Steatohepatitis (NASH)
Cilofexor has been extensively studied for its efficacy in treating NASH, a condition characterized by fat accumulation in the liver that can lead to cirrhosis and liver failure.
- Phase 2 Trials : A double-blind, placebo-controlled trial involving 140 patients demonstrated that this compound significantly reduced hepatic steatosis as measured by magnetic resonance imaging-proton density fat fraction (MRI-PDFF). Patients receiving 100 mg of this compound exhibited a median relative decrease in MRI-PDFF of -22.7% compared to an increase of 1.9% in the placebo group (P = 0.003) .
- Biochemical Improvements : Significant reductions were also observed in liver biochemistry markers, including serum gamma-glutamyltransferase and primary bile acids .
Study | Dose | Median Relative Decrease in MRI-PDFF | P-Value |
---|---|---|---|
Phase 2 Trial | 100 mg | -22.7% | 0.003 |
Phase 2 Trial | 30 mg | -1.8% | 0.17 |
Primary Sclerosing Cholangitis (PSC)
This compound has shown promise in treating PSC, a chronic liver disease characterized by inflammation and scarring of the bile ducts.
- Safety and Efficacy : In a separate phase II study involving patients with large-duct PSC, this compound led to significant improvements in liver biochemistry markers such as alkaline phosphatase and alanine aminotransferase . The study found that this compound at a dose of 100 mg resulted in a median reduction of serum alkaline phosphatase by -21% (P = 0.029) .
Study | Dose | Median Reduction in Alkaline Phosphatase | P-Value |
---|---|---|---|
Phase II Study | 100 mg | -21% | 0.029 |
Phase II Study | 30 mg | -20% (not significant) | N/A |
Case Study: Efficacy in NASH
In a clinical setting, one patient treated with this compound for NASH exhibited notable improvements after 24 weeks. The patient had a baseline MRI-PDFF of 15%, which decreased to approximately 10% following treatment, alongside significant reductions in liver enzymes .
Case Study: Tolerability in PSC
Another case involved a patient with PSC who received this compound over a period of 12 weeks. The patient reported mild adverse effects but showed marked improvement in biochemical markers, leading to discussions about long-term treatment options .
Mechanism of Action
Cilofexor exerts its effects by activating the farnesoid X receptor, a nuclear receptor that regulates bile acid, lipid, and cholesterol metabolism. The binding of this compound to the farnesoid X receptor leads to changes in gene expression, reducing inflammation and fibrosis in the liver. Additionally, this compound may activate other receptors, such as Mas-related G protein-coupled receptor X4 and G protein-coupled bile acid receptor 1, contributing to its therapeutic effects .
Comparison with Similar Compounds
Cilofexor vs. Obeticholic Acid (OCA)
Mechanistic Differences :
This compound vs. Px-104 (First-Generation FXR Agonist)
Key Insight : Px-104 induced marked LDL-C and HDL-C reductions in mice, likely due to hepatic FXR activation, whereas this compound’s intestinally biased profile preserved lipid stability .
This compound vs. Tropifexor
Data Tables
Table 1: Phase II Clinical Outcomes in NASH
Compound | Trial Duration | MRI-PDFF Reduction | ALT Reduction | Pruritus Incidence |
---|---|---|---|---|
This compound | 24 weeks | -22.7%* | -20.5 U/L | 14% (100 mg) |
Obeticholic Acid | 18 months | -21.8% | -23 U/L | 28% |
Table 2: Pharmacokinetic Interactions
Key Challenges :
- Balancing systemic FXR activation for fibrosis reduction without exacerbating pruritus.
- Addressing drug-drug interactions with OATP/BCRP inhibitors (e.g., rifampin) .
This compound remains a promising candidate for NASH, pending further trials optimizing dosing and patient stratification.
Biological Activity
Cilofexor, also known as GS-9674, is a selective farnesoid X receptor (FXR) agonist that has garnered attention for its potential therapeutic applications in liver diseases, particularly non-alcoholic steatohepatitis (NASH) and primary sclerosing cholangitis (PSC). This article delves into the biological activity of this compound, highlighting its pharmacodynamics, clinical efficacy, safety profile, and ongoing research findings.
This compound functions primarily as an FXR agonist. FXR is a nuclear receptor that plays a crucial role in regulating bile acid, lipid, and glucose metabolism. By activating FXR, this compound influences various metabolic pathways, which can lead to beneficial effects in liver diseases characterized by cholestasis and fibrosis.
Key Biological Activities
- Bile Acid Regulation : this compound has been shown to reduce serum bile acids and the bile acid intermediate 7α-hydroxy-4-cholesten-3-one (C4) in a dose-dependent manner .
- Fibrosis Improvement : Clinical studies indicate that this compound may improve liver fibrosis in patients with advanced liver diseases .
- Lipid Metabolism : By modulating FXR activity, this compound can potentially reduce hepatic steatosis and improve lipid profiles .
Phase 1 Studies
In a first-in-human study, this compound demonstrated favorable pharmacokinetics with high protein binding (>99%) and a bi-exponential disposition profile. The study involved 120 participants and assessed the drug's safety and tolerability over multiple dosing regimens . Results indicated that this compound effectively increased plasma levels of FGF19 while reducing bile acid levels.
Phase 1b Study in PSC Patients
A proof-of-concept phase 1b study evaluated this compound's safety and efficacy in patients with compensated cirrhosis due to PSC. The study administered escalating doses (30 mg to 100 mg) over 12 weeks and found significant improvements in markers of cholestasis and liver injury without exacerbating common side effects associated with FXR agonists .
NASH Patient Trials
In a double-blind, placebo-controlled phase 2 trial involving patients with noncirrhotic NASH, this compound was administered at doses of 30 mg and 100 mg. The results showed a significant reduction in hepatic steatosis measured by MRI-PDFF, with the 100 mg group exhibiting a median relative decrease of -22.7% compared to placebo . Additionally, serum markers such as gamma-glutamyltransferase and primary bile acids decreased significantly.
Combination Therapy Studies
A phase 2b trial explored combination therapies including this compound with firsocostat for patients with bridging fibrosis or compensated cirrhosis. Results indicated that the combination therapy was well tolerated and led to improvements in NASH activity scores and reductions in hepatic fibrosis markers .
Summary of Clinical Findings
Study Type | Population | Dosage | Key Findings |
---|---|---|---|
Phase 1 | Healthy volunteers | Escalating doses | Increased FGF19; reduced C4; well tolerated |
Phase 1b | PSC patients | 30 mg - 100 mg | Improved cholestasis markers; safe |
Phase 2 | Noncirrhotic NASH | 30 mg / 100 mg | Significant reduction in hepatic steatosis; safe |
Phase 2b | Bridging fibrosis/cirrhosis | Combination therapy | Improved NASH scores; well tolerated |
Safety Profile
This compound has demonstrated a manageable safety profile across various studies. Common adverse effects include moderate to severe pruritus, particularly at higher doses (14% in the 100 mg group) compared to placebo . Importantly, no new safety issues were identified even in extended studies lasting up to 96 weeks .
Q & A
Basic Research Questions
Q. What experimental models are most suitable for evaluating cilofexor’s mechanism of action as a nonsteroidal FXR agonist?
Methodological Answer: Preclinical studies often utilize rodent models (e.g., high-fat diet-induced NASH mice) to assess this compound’s anti-inflammatory and antifibrotic effects via transcriptional profiling of FXR target genes (e.g., Fgf15, Cyp7a1). For intestinal bias evaluation, comparative gene expression analysis in liver vs. intestinal tissues is critical . Humanized FXR transgenic models or primary hepatocyte cultures further validate tissue-specific responses.
Q. How should dose-escalation studies be designed to balance efficacy and safety in early-phase trials?
Methodological Answer: Phase I trials typically employ staggered cohorts with single-ascending (SAD) and multiple-ascending (MAD) dose protocols. Safety endpoints (e.g., ALT levels, lipid profiles) are monitored alongside pharmacokinetic (PK) parameters like Cmax and AUC. Blinded safety reviews between dosing periods ensure risk mitigation .
Q. What biomarkers are validated for monitoring this compound’s pharmacodynamic (PD) effects in clinical trials?
Methodological Answer: Serum bile acids (e.g., 7α-hydroxy-4-cholesten-3-one), FGF19, and liver stiffness measurements (via transient elastography) are primary PD markers. In NASH trials, histopathological scoring (e.g., NAFLD Activity Score) and collagen-specific imaging biomarkers are used .
Advanced Research Questions
Q. How can conflicting data on this compound’s hepatic vs. intestinal FXR activation be reconciled?
Methodological Answer: Mechanistic discrepancies arise from tissue-specific FXR signaling. Fluorescent imaging in hepatoma cells reveals this compound’s shorter membrane residence time compared to other FXR agonists (e.g., Px-102), favoring intestinal over hepatic activation. Rodent studies with intestinal-specific FXR knockouts can isolate pathways .
Q. What statistical approaches address heterogeneity in this compound’s antifibrotic efficacy across patient subgroups?
Methodological Answer: Stratified randomization by baseline fibrosis stage (e.g., F0-F4) and covariate-adjusted mixed-effects models account for variability. Longitudinal analyses (e.g., repeated liver biopsies) combined with machine learning (e.g., clustering of transcriptomic data) identify responder/non-responder phenotypes .
Q. How do this compound’s PK/PD properties influence trial design for chronic liver diseases like PSC?
Methodological Answer: Population PK modeling integrates covariates (e.g., cholestasis severity, CYP3A4 polymorphisms) to predict exposure-response relationships. Adaptive trial designs with interim futility analyses optimize dosing regimens for long-term outcomes (e.g., transplant-free survival) .
Q. Data Analysis and Reproducibility
Q. What strategies ensure reproducibility in this compound’s preclinical efficacy data?
Methodological Answer: Standardized protocols for animal husbandry (e.g., diet, circadian timing) and blinded histopathological assessments are essential. Public deposition of raw RNA-seq data (e.g., GEO database) and detailed methods in supplementary materials enhance transparency .
Q. How should contradictory findings between rodent and primate models be addressed?
Methodological Answer: Cross-species comparative studies (e.g., hepatic FXR occupancy assays) and in vitro organoid models validate translatability. Meta-analyses of interspecies PK/PD correlations adjust for metabolic differences (e.g., bile acid composition) .
Q. Tables of Key Data
Parameter | Preclinical (Rodent) | Clinical (Phase II) | Source |
---|---|---|---|
EC50 (FXR activation) | 43 nM | N/A | |
Reduction in Liver Collagen | 40-50% | 25-30% (NASH patients) | |
ALT Elevation Risk | Minimal | Dose-dependent |
Properties
IUPAC Name |
2-[3-[2-chloro-4-[[5-cyclopropyl-3-(2,6-dichlorophenyl)-1,2-oxazol-4-yl]methoxy]phenyl]-3-hydroxyazetidin-1-yl]pyridine-4-carboxylic acid | |
---|---|---|
Source | PubChem | |
URL | https://pubchem.ncbi.nlm.nih.gov | |
Description | Data deposited in or computed by PubChem | |
InChI |
InChI=1S/C28H22Cl3N3O5/c29-20-2-1-3-21(30)24(20)25-18(26(39-33-25)15-4-5-15)12-38-17-6-7-19(22(31)11-17)28(37)13-34(14-28)23-10-16(27(35)36)8-9-32-23/h1-3,6-11,15,37H,4-5,12-14H2,(H,35,36) | |
Source | PubChem | |
URL | https://pubchem.ncbi.nlm.nih.gov | |
Description | Data deposited in or computed by PubChem | |
InChI Key |
KZSKGLFYQAYZCO-UHFFFAOYSA-N | |
Source | PubChem | |
URL | https://pubchem.ncbi.nlm.nih.gov | |
Description | Data deposited in or computed by PubChem | |
Canonical SMILES |
C1CC1C2=C(C(=NO2)C3=C(C=CC=C3Cl)Cl)COC4=CC(=C(C=C4)C5(CN(C5)C6=NC=CC(=C6)C(=O)O)O)Cl | |
Source | PubChem | |
URL | https://pubchem.ncbi.nlm.nih.gov | |
Description | Data deposited in or computed by PubChem | |
Molecular Formula |
C28H22Cl3N3O5 | |
Source | PubChem | |
URL | https://pubchem.ncbi.nlm.nih.gov | |
Description | Data deposited in or computed by PubChem | |
Molecular Weight |
586.8 g/mol | |
Source | PubChem | |
URL | https://pubchem.ncbi.nlm.nih.gov | |
Description | Data deposited in or computed by PubChem | |
CAS No. |
1418274-28-8 | |
Record name | Cilofexor [INN] | |
Source | ChemIDplus | |
URL | https://pubchem.ncbi.nlm.nih.gov/substance/?source=chemidplus&sourceid=1418274288 | |
Description | ChemIDplus is a free, web search system that provides access to the structure and nomenclature authority files used for the identification of chemical substances cited in National Library of Medicine (NLM) databases, including the TOXNET system. | |
Record name | Cilofexor | |
Source | DrugBank | |
URL | https://www.drugbank.ca/drugs/DB15168 | |
Description | The DrugBank database is a unique bioinformatics and cheminformatics resource that combines detailed drug (i.e. chemical, pharmacological and pharmaceutical) data with comprehensive drug target (i.e. sequence, structure, and pathway) information. | |
Explanation | Creative Common's Attribution-NonCommercial 4.0 International License (http://creativecommons.org/licenses/by-nc/4.0/legalcode) | |
Record name | CILOFEXOR | |
Source | FDA Global Substance Registration System (GSRS) | |
URL | https://gsrs.ncats.nih.gov/ginas/app/beta/substances/YUN2306954 | |
Description | The FDA Global Substance Registration System (GSRS) enables the efficient and accurate exchange of information on what substances are in regulated products. Instead of relying on names, which vary across regulatory domains, countries, and regions, the GSRS knowledge base makes it possible for substances to be defined by standardized, scientific descriptions. | |
Explanation | Unless otherwise noted, the contents of the FDA website (www.fda.gov), both text and graphics, are not copyrighted. They are in the public domain and may be republished, reprinted and otherwise used freely by anyone without the need to obtain permission from FDA. Credit to the U.S. Food and Drug Administration as the source is appreciated but not required. | |
Disclaimer and Information on In-Vitro Research Products
Please be aware that all articles and product information presented on BenchChem are intended solely for informational purposes. The products available for purchase on BenchChem are specifically designed for in-vitro studies, which are conducted outside of living organisms. In-vitro studies, derived from the Latin term "in glass," involve experiments performed in controlled laboratory settings using cells or tissues. It is important to note that these products are not categorized as medicines or drugs, and they have not received approval from the FDA for the prevention, treatment, or cure of any medical condition, ailment, or disease. We must emphasize that any form of bodily introduction of these products into humans or animals is strictly prohibited by law. It is essential to adhere to these guidelines to ensure compliance with legal and ethical standards in research and experimentation.