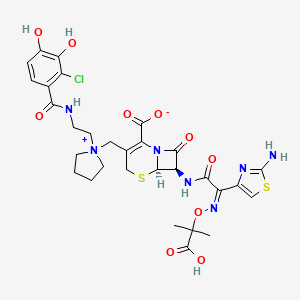
Cefiderocol
- Click on QUICK INQUIRY to receive a quote from our team of experts.
- With the quality product at a COMPETITIVE price, you can focus more on your research.
Overview
Description
Cefiderocol is a novel siderophore cephalosporin antibiotic designed to combat multidrug-resistant Gram-negative bacterial infections. It was first approved by the US FDA in 2019 for the treatment of complicated urinary tract infections and later for hospital-acquired bacterial pneumonia and ventilator-associated bacterial pneumonia . This compound’s unique mechanism of action involves binding to extracellular iron and utilizing bacterial iron transport systems to penetrate the bacterial cell wall .
Preparation Methods
Synthetic Routes and Reaction Conditions: Cefiderocol is synthesized through a multi-step process involving the formation of a cephalosporin core and the attachment of a catechol-type siderophore moiety. The key steps include:
- Formation of the cephalosporin core through a series of condensation and cyclization reactions.
- Introduction of the catechol siderophore moiety via a coupling reaction with the cephalosporin core.
- Purification and crystallization to obtain the final product .
Industrial Production Methods: Industrial production of this compound involves large-scale synthesis using optimized reaction conditions to ensure high yield and purity. The process includes:
- Bulk synthesis of the cephalosporin core and siderophore moiety.
- Coupling of the two components under controlled conditions.
- Purification using chromatography and crystallization techniques.
- Quality control to ensure compliance with pharmaceutical standards .
Chemical Reactions Analysis
Biochemical Interaction with Pseudomonas aeruginosa PBP3
Cefiderocol reacts with penicillin-binding protein 3 (PBP3) in P. aeruginosa through a mechanism involving covalent binding and side-chain elimination. Kinetic studies reveal:
Compound | kinact (min⁻¹) | KI (µM) | kcat/K (µM⁻¹·min⁻¹) |
---|---|---|---|
This compound | 7.3 ± 0.1 | 7.0 ± 0.1 | 3000 ± 200 |
Ceftazidime | 7.1 ± 0.1 | 6.6 ± 0.1 | 3400 ± 400 |
The reaction proceeds via C3 pyrrolidinium group elimination , forming a stable acyl-enzyme complex (Scheme 1) . Structural analysis shows:
- Salt bridge interactions between this compound’s C7 oxime-carboxylic acid and Arg440 (2.6–3.4 Å) .
- Hydrogen bonding with Thr438 and Glu242 stabilizes the β-lactam ring .
- Unlike cefepime, this compound’s chlorocatechol side chain enhances siderophore-mediated uptake but does not directly participate in PBP3 binding .
Degradation Pathways and Metabolite Formation
This compound undergoes limited enzymatic degradation but forms stable metabolites in plasma:
Metabolite | Plasma AUC Contribution (%) |
---|---|
This compound | 92.3 |
Pyrrolidine chlorobenzamide (PCBA) | 4.7 |
Minor metabolites | < 2 each |
PCBA arises from hydrolytic cleavage of the C3 side chain, a degradation pathway confirmed via 14C-labelled studies . The terminal elimination half-life is 2–3 hours, with renal excretion as the primary route .
Analytical Characterization via LC-ITD-MS/MS
Chromatographic and mass spectrometric parameters for this compound analysis include:
Mass Transitions
Analyte | Retention Time (min) | Precursor Ion (m/z) | Product Ion (m/z) |
---|---|---|---|
This compound | 1.58 | 752.1 | 285.2 |
This compound-d12 | 1.58 | 764.1 | 297.3 |
Gradient Profile
Time (min) | Mobile Phase A (%) | Mobile Phase B (%) | Flow Rate (mL/min) |
---|---|---|---|
0 | 95 | 5 | 0.5 |
2 | 5 | 95 | 0.5 |
This method achieves baseline separation within 4 minutes, demonstrating this compound’s stability under acidic mobile phase conditions .This compound’s reactivity is defined by its targeted PBP3 binding, controlled degradation into PCBA, and robust analytical detectability. These properties underpin its clinical efficacy against multidrug-resistant Gram-negative pathogens.
Scientific Research Applications
Cefiderocol has a wide range of applications in scientific research, including:
Chemistry: Used as a model compound to study siderophore-mediated iron transport and β-lactamase resistance mechanisms
Biology: Investigated for its role in bacterial iron metabolism and its impact on bacterial growth and virulence
Medicine: Extensively studied for its efficacy against multidrug-resistant Gram-negative bacterial infections, including those caused by carbapenem-resistant Enterobacterales, Pseudomonas aeruginosa, and Acinetobacter baumannii
Industry: Utilized in the development of new antibiotics and as a reference compound in antimicrobial susceptibility testing
Mechanism of Action
Cefiderocol is structurally and functionally similar to other cephalosporins, such as cefepime and ceftazidime, but with unique features:
Cefepime: Like this compound, cefepime has a pyrrolidinium group that enhances stability against β-lactamases. .
Ceftazidime: Both this compound and ceftazidime are effective against Gram-negative bacteria, but this compound’s siderophore activity offers enhanced penetration and efficacy against multidrug-resistant strains
Unique Features:
Siderophore Moiety: this compound’s ability to utilize bacterial iron transport systems sets it apart from other cephalosporins.
Broad Spectrum of Activity: this compound is effective against a wide range of multidrug-resistant Gram-negative bacteria
Comparison with Similar Compounds
- Cefepime
- Ceftazidime
- Meropenem
- Imipenem .
Cefiderocol represents a significant advancement in the fight against multidrug-resistant bacterial infections, offering a unique mechanism of action and broad-spectrum efficacy. Its development and application continue to be a focus of scientific research and clinical practice.
Biological Activity
Cefiderocol, a novel siderophore cephalosporin, has emerged as a critical agent in the fight against multidrug-resistant (MDR) Gram-negative bacteria. Its unique mechanism of action, combined with its stability against various beta-lactamases, makes it a promising candidate for treating severe infections caused by resistant pathogens. This article delves into the biological activity of this compound, supported by data tables, case studies, and detailed research findings.
This compound operates through a unique mechanism that enhances its ability to penetrate the cell membranes of Gram-negative bacteria. It utilizes iron transport systems to gain entry into bacterial cells. By binding to ferric iron via its catechol moiety, this compound effectively mimics natural siderophores, allowing it to bypass typical resistance mechanisms employed by bacteria.
Antimicrobial Activity
This compound has demonstrated potent antimicrobial activity against a wide range of MDR Gram-negative pathogens. Below is a summary of its activity based on various studies:
Pathogen | MIC90 (mg/L) | Comparison Antibiotics |
---|---|---|
Pseudomonas aeruginosa | 0.5 | Meropenem: 8, Ceftazidime: 4 |
Klebsiella pneumoniae | 1 | Meropenem: >16 |
Acinetobacter baumannii | 0.5 | Meropenem: >16 |
Enterobacter cloacae | 1 | Ceftazidime: >16 |
This compound consistently shows lower Minimum Inhibitory Concentration (MIC) values than comparator antibiotics across various studies, indicating its superior efficacy against resistant strains .
Efficacy Against Biofilms
One notable aspect of this compound's activity is its effectiveness against biofilms formed by MDR pathogens. A study reported that this compound achieved a 93% reduction in biofilm levels of Pseudomonas aeruginosa, significantly outperforming other antibiotics such as imipenem and tobramycin, which showed reductions between 49% and 82% . This characteristic is particularly advantageous in clinical settings where biofilm-associated infections are prevalent.
Case Study 1: Treatment of Invasive Infections
A clinical report highlighted the successful use of this compound as monotherapy for invasive infections caused by MDR Acinetobacter baumannii and Klebsiella pneumoniae. The study noted that patients exhibited significant clinical improvement and microbiological clearance post-treatment, underscoring this compound's potential as an effective treatment option for severe infections .
Case Study 2: Pharmacokinetics and Safety
In a pharmacokinetic study involving patients with complicated urinary tract infections, this compound demonstrated favorable pharmacokinetic properties that support its dosing regimen. The safety profile was consistent with prior studies, showing minimal adverse effects while effectively managing infections caused by carbapenem-resistant organisms .
Comparative Studies
Recent investigations have compared this compound's activity against various beta-lactamase producing strains. A study involving 189 non-fermentative Gram-negative bacteria found that this compound maintained potent activity against all tested strains, including those producing metallo-beta-lactamases (MBLs), where it exhibited MIC values significantly lower than those observed for other beta-lactams .
Q & A
Q. Basic: How should researchers design in vitro studies to assess cefiderocol’s activity against multidrug-resistant (MDR) Gram-negative pathogens?
Methodological Answer:
- Key Parameters : Include standardized broth microdilution under iron-depleted conditions to mimic host physiology, as this compound’s siderophore activity is iron concentration-dependent .
- Control Strains : Use reference strains (e.g., Acinetobacter baumannii ATCC 19606) and clinical isolates with defined resistance mechanisms (e.g., carbapenemases, extended-spectrum β-lactamases) to contextualize MIC values .
- Iron Modulation : Test activity across varying iron concentrations (e.g., 0–100 μM) to quantify the "Trojan horse" effect .
- Reproducibility : Follow CLSI/EUCAST guidelines for susceptibility testing and report iron chelator usage (e.g., deferoxamine) to ensure comparability across studies .
Q. Basic: What pharmacokinetic/pharmacodynamic (PK/PD) parameters are critical for preclinical modeling of this compound efficacy?
Methodological Answer:
- Time-Dependent Activity : Focus on the time above MIC (fT > MIC) in serum and tissue, as this compound exhibits concentration-independent bactericidal activity .
- Protein Binding : Adjust for ~60% protein binding in plasma to calculate free drug concentrations .
- Iron Saturation : Model iron dynamics in infection sites (e.g., urinary tract, lungs) to predict drug penetration via siderophore-mediated uptake .
- Animal Models : Use neutropenic murine thigh or lung infection models with iron supplementation to mimic human pharmacokinetics .
Q. Advanced: How can researchers reconcile contradictory mortality data between randomized trials (e.g., CREDIBLE-CR) and observational studies (e.g., PROVE)?
Methodological Answer:
- Confounder Adjustment : Apply inverse probability of treatment weighting (IPTW) or propensity score matching to balance baseline characteristics (e.g., comorbidities, pathogen resistance profiles) in observational cohorts .
- Endpoint Harmonization : Align definitions of "clinical cure" and "mortality" across studies (e.g., 30-day all-cause vs. infection-related mortality) .
- Subgroup Analysis : Stratify by infection type (e.g., bacteremia vs. pneumonia) and pathogen (e.g., A. baumannii vs. P. aeruginosa) to identify context-specific efficacy .
- Meta-Analysis : Pool data from Phase 2/3 trials and real-world studies using random-effects models to quantify heterogeneity .
Q. Advanced: What experimental approaches are recommended to evaluate the emergence of this compound resistance during treatment?
Methodological Answer:
- Serial Isolate Collection : Perform whole-genome sequencing on pre- and post-treatment isolates to detect mutations in siderophore receptors (e.g., pirA), β-lactamases, or porin channels .
- Chequerboard Assays : Test for synergy/antagonism with β-lactams, aminoglycosides, or tetracyclines to identify resistance-suppressing combinations .
- Dynamic Resistance Models : Use hollow-fiber infection systems to simulate human PK/PD and monitor MIC shifts under prolonged drug exposure .
- Resistance Gene Screening : Employ PCR for metallo-β-lactamases (e.g., NDM, VIM) and efflux pump overexpression .
Q. Advanced: How should clinical trials be designed to compare this compound monotherapy versus combination regimens for carbapenem-resistant infections?
Methodological Answer:
- Adaptive Design : Use a master protocol with Bayesian statistics to allocate patients to monotherapy or combination arms based on interim efficacy/safety data .
- Endpoint Selection : Prioritize composite endpoints (e.g., microbiological eradication + clinical cure) to capture multifaceted outcomes .
- Standardized Combinations : Test this compound with agents lacking overlapping resistance mechanisms (e.g., colistin, fosfomycin) and predefine dosing protocols .
- Rescue Therapy Criteria : Define thresholds for regimen escalation (e.g., persistent bacteremia at 72 hours) to maintain ethical rigor .
Q. Basic: What statistical methods are appropriate for analyzing microbiological clearance in this compound trials?
Methodological Answer:
- Survival Analysis : Use Kaplan-Meier curves with log-rank tests to compare time-to-clearance between treatment arms .
- Multivariate Regression : Adjust for covariates (e.g., baseline MIC, renal function) via Cox proportional hazards models .
- Missing Data Handling : Apply multiple imputation or worst-case sensitivity analysis for missing blood culture follow-ups .
Q. Advanced: How can real-world evidence (RWE) studies complement this compound clinical trial data?
Methodological Answer:
- Data Harmonization : Use common data models (e.g., OMOP) to standardize variables across electronic health records and trial databases .
- Bias Mitigation : Apply target trial emulation frameworks to minimize confounding by indication in retrospective cohorts .
- Outcome Validation : Cross-reference RWE findings with trial subpopulations (e.g., ICU patients, carbapenem-resistant A. baumannii) .
Q. Basic: What protocols ensure reproducibility in in vivo models of this compound efficacy?
Methodological Answer:
- Detailed Methodology : Report strain origins, inoculation doses, and iron supplementation levels in animal feed .
- Blinding : Use coded treatment groups to reduce observer bias in outcome assessments .
- Sample Size Justification : Perform power calculations based on pilot data for primary endpoints (e.g., bacterial load reduction) .
Q. Advanced: What molecular mechanisms underlie this compound resistance, and how can they be experimentally validated?
Methodological Answer:
- Mechanistic Studies :
- Siderophore Receptor Knockouts : Use CRISPR-Cas9 to delete pirA or cirA in A. baumannii and assess MIC changes .
- Efflux Pump Inhibition : Test phenylalanine-arginine β-naphthylamide (PAβN) to quantify efflux contributions .
- Enzyme Hydrolysis Assays : Measure this compound degradation by purified β-lactamases (e.g., OXA-23, KPC) .
Q. Basic: How should researchers validate susceptibility testing methods for this compound in clinical isolates?
Methodological Answer:
- Comparative Methods : Cross-validate disc diffusion, E-test, and broth microdilution against EUCAST/CLSI breakpoints (MIC ≤2 μg/mL) .
- Iron Chelation : Supplement media with deferoxamine (50 μg/mL) to replicate in vivo iron-limited conditions .
- Quality Control : Include reference strains (e.g., E. coli ATCC 25922) in each assay run .
Properties
Key on ui mechanism of action |
Cefiderocol acts by binding to and inhibiting penicillin-binding proteins (PBPs), preventing cell wall synthesis and ultimately causing death of the bacterial cell. Like other β-lactam antibiotics cefiderocol is able to enter bacterial cells via passive diffusion through porins. Unlike other β-lactams, cefiderocol contains a chlorocatechol group which allows it to chelate iron. Once bound to ferric iron cefiderocol is able to undergo active transport into bacterial cells through iron channels in the outer cell membrane such as those encoded by the *cirA* and *fiu* genes in *E. coli* or the *PiuA* gene in *P. aeruginosa*. Once inside the cell, cefiderocol binds to and inhibits PBP3 with high affinity thereby preventing the linking of peptodoglycan layers via the pentapeptide bridge. PBP1a, 1b, 2,and 4 are also bound and inhibited by cefiderocol but with a lesser potency than PBP3 and are therefore expected to contribute less to its antibacterial effect. |
---|---|
CAS No. |
1225208-94-5 |
Molecular Formula |
C30H34ClN7O10S2 |
Molecular Weight |
752.2 g/mol |
IUPAC Name |
(6R,7R)-7-[[(2E)-2-(2-amino-1,3-thiazol-4-yl)-2-(2-carboxypropan-2-yloxyimino)acetyl]amino]-3-[[1-[2-[(2-chloro-3,4-dihydroxybenzoyl)amino]ethyl]pyrrolidin-1-ium-1-yl]methyl]-8-oxo-5-thia-1-azabicyclo[4.2.0]oct-2-ene-2-carboxylate |
InChI |
InChI=1S/C30H34ClN7O10S2/c1-30(2,28(46)47)48-36-19(16-13-50-29(32)34-16)24(42)35-20-25(43)37-21(27(44)45)14(12-49-26(20)37)11-38(8-3-4-9-38)10-7-33-23(41)15-5-6-17(39)22(40)18(15)31/h5-6,13,20,26H,3-4,7-12H2,1-2H3,(H7-,32,33,34,35,36,39,40,41,42,44,45,46,47)/t20-,26-/m1/s1 |
InChI Key |
DBPPRLRVDVJOCL-FQRUVTKNSA-N |
SMILES |
CC(C)(C(=O)O)ON=C(C1=CSC(=N1)N)C(=O)NC2C3N(C2=O)C(=C(CS3)C[N+]4(CCCC4)CCNC(=O)C5=C(C(=C(C=C5)O)O)Cl)C(=O)[O-] |
Isomeric SMILES |
CC(C)(C(=O)O)O/N=C(\C1=CSC(=N1)N)/C(=O)N[C@H]2[C@@H]3N(C2=O)C(=C(CS3)C[N+]4(CCCC4)CCNC(=O)C5=C(C(=C(C=C5)O)O)Cl)C(=O)[O-] |
Canonical SMILES |
CC(C)(C(=O)O)ON=C(C1=CSC(=N1)N)C(=O)NC2C3N(C2=O)C(=C(CS3)C[N+]4(CCCC4)CCNC(=O)C5=C(C(=C(C=C5)O)O)Cl)C(=O)[O-] |
Appearance |
Solid powder |
Purity |
>95% (or refer to the Certificate of Analysis5 |
shelf_life |
>2 years if stored properly |
solubility |
Soluble in DMSO, not in water |
storage |
Dry, dark and at 0 - 4 C for short term (days to weeks) or -20 C for long term (months to years). |
Synonyms |
S-649266; S 649266; S649266; GSK2696266D; GSK-2696266D; GSK 2696266D; S-649266D; S 649266D; S649266D; Cefiderocol; Fetroja. |
Origin of Product |
United States |
Disclaimer and Information on In-Vitro Research Products
Please be aware that all articles and product information presented on BenchChem are intended solely for informational purposes. The products available for purchase on BenchChem are specifically designed for in-vitro studies, which are conducted outside of living organisms. In-vitro studies, derived from the Latin term "in glass," involve experiments performed in controlled laboratory settings using cells or tissues. It is important to note that these products are not categorized as medicines or drugs, and they have not received approval from the FDA for the prevention, treatment, or cure of any medical condition, ailment, or disease. We must emphasize that any form of bodily introduction of these products into humans or animals is strictly prohibited by law. It is essential to adhere to these guidelines to ensure compliance with legal and ethical standards in research and experimentation.