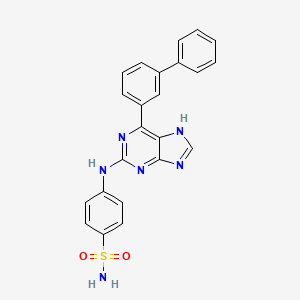
CDK2 inhibitor 73
- Click on QUICK INQUIRY to receive a quote from our team of experts.
- With the quality product at a COMPETITIVE price, you can focus more on your research.
Overview
Description
CDK2-IN-4 is a highly selective inhibitor of cyclin-dependent kinase 2 (CDK2). Cyclin-dependent kinase 2 is a crucial enzyme involved in the regulation of the cell cycle, particularly in the transition from the G1 phase to the S phase. CDK2-IN-4 has shown significant potential in cancer research due to its ability to selectively inhibit CDK2, thereby preventing uncontrolled cell proliferation, which is a hallmark of cancer .
Mechanism of Action
Target of Action
The primary target of CDK2 inhibitor 73, also known as CDK2-IN-4, is Cyclin-dependent kinase 2 (CDK2) . CDK2 is a member of the larger cell cycle regulating CDK family of kinases, activated by binding partner cyclins . It plays a critical role in controlling the G1 to S-phase transition and promotes DNA replication during the cell cycle .
Mode of Action
This compound interacts with its target, CDK2, by binding to an allosteric pocket . This interaction results in the suppression of the Thr160 activating phosphorylation on CDK2 . It also leads to the downregulation of cyclin E1 , which is a key regulator of CDK2 .
Biochemical Pathways
The interaction of this compound with CDK2 affects the cell cycle regulation pathway . Specifically, it disrupts the CDK2/cyclin E1 and CDK2/cyclin A2 complexes , thereby inhibiting the phosphorylation of Rb (pRb) and inducing G1 cell cycle arrest . This results in the blockage of the G1/S transition and impedes DNA replication .
Result of Action
The action of this compound leads to several molecular and cellular effects. It causes a rapid loss of substrate phosphorylation . The inhibitor also induces G1 cell cycle arrest , thereby impeding cell proliferation . Furthermore, it downregulates cyclin E1 and suppresses activating phosphorylation of CDK2 on Thr160 .
Action Environment
The efficacy and stability of this compound can be influenced by various environmental factors. For instance, the overexpression of cyclin E1 in certain cancer cells can predict the dependency on CDK2 and thus the sensitivity to CDK2 inhibition . Moreover, the compound shows promise as a monotherapy and combination therapy, suggesting its action can be influenced by the presence of other drugs .
Biochemical Analysis
Biochemical Properties
CDK2 Inhibitor 73 interacts with CDK2, a serine/threonine kinase that forms a complex with cyclin E to regulate the G1/S transition and promote DNA replication during the cell cycle . The compound exhibits high potency towards CDK2, but is significantly less active towards CDK1 . This selectivity makes this compound a valuable tool for studies of cell cycle regulation .
Cellular Effects
This compound has been shown to have significant effects on various types of cells and cellular processes. It can influence cell function by impacting cell signaling pathways, gene expression, and cellular metabolism . For instance, in cancer cells with CCNE1 amplification or overexpression, this compound can induce G1 growth arrest and control tumor growth .
Molecular Mechanism
The molecular mechanism of action of this compound involves binding interactions with biomolecules, enzyme inhibition, and changes in gene expression . The compound binds to CDK2, inhibiting its activity and thereby disrupting the normal progression of the cell cycle . This results in the arrest of cell proliferation, which is a key factor in the growth of tumors .
Temporal Effects in Laboratory Settings
In laboratory settings, the effects of this compound have been observed to change over time . The compound exhibits stability and does not degrade rapidly, making it suitable for long-term studies . It has been shown to have long-term effects on cellular function in both in vitro and in vivo studies .
Dosage Effects in Animal Models
In animal models, the effects of this compound have been found to vary with different dosages . At lower doses, the compound effectively controls tumor growth, while at higher doses, it may cause toxic or adverse effects . This highlights the importance of carefully determining the optimal dosage for therapeutic applications .
Metabolic Pathways
This compound is involved in the regulation of several metabolic pathways. It interacts with enzymes and cofactors that are part of the cell cycle regulation pathway . The compound can also affect metabolic flux and metabolite levels, further influencing cellular function .
Transport and Distribution
Given its role in cell cycle regulation, it is likely that the compound interacts with various transporters or binding proteins, which could affect its localization or accumulation within cells .
Subcellular Localization
It is plausible that the compound may be directed to specific compartments or organelles within the cell due to targeting signals or post-translational modifications . Understanding the subcellular localization of this compound could provide further insights into its activity and function .
Preparation Methods
The synthesis of CDK2-IN-4 involves several steps, starting with the preparation of the core structure, followed by the introduction of various substituents to enhance its inhibitory activity. One common synthetic route involves the use of pyrazolo[3,4-d]pyrimidine derivatives. The reaction conditions typically include the use of organic solvents, catalysts, and controlled temperatures to ensure high yield and purity . Industrial production methods may involve large-scale synthesis using similar reaction conditions but optimized for efficiency and cost-effectiveness .
Chemical Reactions Analysis
CDK2-IN-4 undergoes several types of chemical reactions, including:
Oxidation: This reaction can modify the functional groups on the compound, potentially altering its inhibitory activity.
Reduction: This reaction can be used to reduce specific functional groups, affecting the compound’s stability and reactivity.
Substitution: Common reagents for substitution reactions include halogens and nucleophiles, which can replace specific atoms or groups in the compound.
Major Products: The major products formed from these reactions depend on the specific reagents and conditions used. .
Scientific Research Applications
CDK2-IN-4 has a wide range of scientific research applications, including:
Chemistry: Used as a tool compound to study the role of CDK2 in various chemical reactions and pathways.
Biology: Helps in understanding the biological processes regulated by CDK2, such as cell cycle progression and DNA replication.
Medicine: Investigated for its potential as an anticancer agent due to its ability to inhibit CDK2 and prevent cancer cell proliferation.
Industry: Used in the development of new therapeutic agents targeting CDK2 for various diseases, including cancer .
Comparison with Similar Compounds
CDK2-IN-4 is unique due to its high selectivity for cyclin-dependent kinase 2 over other cyclin-dependent kinases, such as cyclin-dependent kinase 1. This selectivity is crucial for minimizing off-target effects and enhancing therapeutic efficacy. Similar compounds include:
Roscovitine: Another cyclin-dependent kinase inhibitor with broader specificity.
Palbociclib: A selective inhibitor of cyclin-dependent kinase 4 and cyclin-dependent kinase 6, used in breast cancer treatment.
Milciclib: A cyclin-dependent kinase inhibitor with activity against multiple cyclin-dependent kinases, including cyclin-dependent kinase 2 .
CDK2-IN-4 stands out due to its exceptional selectivity and potency against cyclin-dependent kinase 2, making it a valuable tool in cancer research and potential therapeutic applications.
Properties
IUPAC Name |
4-[[6-(3-phenylphenyl)-7H-purin-2-yl]amino]benzenesulfonamide |
Source
|
---|---|---|
Source | PubChem | |
URL | https://pubchem.ncbi.nlm.nih.gov | |
Description | Data deposited in or computed by PubChem | |
InChI |
InChI=1S/C23H18N6O2S/c24-32(30,31)19-11-9-18(10-12-19)27-23-28-20(21-22(29-23)26-14-25-21)17-8-4-7-16(13-17)15-5-2-1-3-6-15/h1-14H,(H2,24,30,31)(H2,25,26,27,28,29) |
Source
|
Source | PubChem | |
URL | https://pubchem.ncbi.nlm.nih.gov | |
Description | Data deposited in or computed by PubChem | |
InChI Key |
FUGRWXRQJGJIER-UHFFFAOYSA-N |
Source
|
Source | PubChem | |
URL | https://pubchem.ncbi.nlm.nih.gov | |
Description | Data deposited in or computed by PubChem | |
Canonical SMILES |
C1=CC=C(C=C1)C2=CC(=CC=C2)C3=C4C(=NC(=N3)NC5=CC=C(C=C5)S(=O)(=O)N)N=CN4 |
Source
|
Source | PubChem | |
URL | https://pubchem.ncbi.nlm.nih.gov | |
Description | Data deposited in or computed by PubChem | |
Molecular Formula |
C23H18N6O2S |
Source
|
Source | PubChem | |
URL | https://pubchem.ncbi.nlm.nih.gov | |
Description | Data deposited in or computed by PubChem | |
Molecular Weight |
442.5 g/mol |
Source
|
Source | PubChem | |
URL | https://pubchem.ncbi.nlm.nih.gov | |
Description | Data deposited in or computed by PubChem | |
Disclaimer and Information on In-Vitro Research Products
Please be aware that all articles and product information presented on BenchChem are intended solely for informational purposes. The products available for purchase on BenchChem are specifically designed for in-vitro studies, which are conducted outside of living organisms. In-vitro studies, derived from the Latin term "in glass," involve experiments performed in controlled laboratory settings using cells or tissues. It is important to note that these products are not categorized as medicines or drugs, and they have not received approval from the FDA for the prevention, treatment, or cure of any medical condition, ailment, or disease. We must emphasize that any form of bodily introduction of these products into humans or animals is strictly prohibited by law. It is essential to adhere to these guidelines to ensure compliance with legal and ethical standards in research and experimentation.