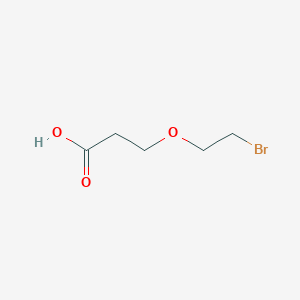
Bromo-PEG1-Acid
Overview
Description
The hydrophilic polyethylene glycol (PEG) linker facilitates solubility in biological applications . This compound is commonly used in bioconjugation and as a building block for the synthesis of small molecules, conjugates of small molecules and/or biomolecules, or other tool compounds for chemical biology and medicinal chemistry .
Mechanism of Action
Target of Action
Bromo-PEG1-Acid is a PEG-based PROTAC linker . The primary targets of this compound are proteins that can be degraded by the Proteolysis Targeting Chimera (PROTAC) technology . The role of these targets varies, but they are typically disease-related proteins that are selectively degraded for therapeutic purposes .
Mode of Action
This compound interacts with its targets through a process called targeted protein degradation . The bromide (Br) group in this compound is a good leaving group for nucleophilic substitution reactions . This allows the compound to bind to its target proteins and induce their degradation . The terminal carboxylic acid can react with primary amine groups in the presence of activators (e.g., EDC, or HATU) to form a stable amide bond .
Biochemical Pathways
The exact biochemical pathways affected by this compound depend on the specific proteins targeted for degradation. The general mechanism involves the ubiquitin-proteasome system, which is responsible for protein degradation in cells . By inducing the degradation of specific proteins, this compound can influence various cellular pathways and processes.
Pharmacokinetics
The hydrophilic peg spacer in the compound is known to increase solubility in aqueous media , which could potentially enhance its bioavailability
Result of Action
The molecular and cellular effects of this compound’s action are primarily related to the degradation of its target proteins . By selectively degrading disease-related proteins, this compound can potentially alter cellular functions and pathways, leading to therapeutic effects. The specific effects would depend on the proteins targeted for degradation.
Action Environment
The action, efficacy, and stability of this compound can be influenced by various environmental factors. For instance, the pH and temperature of the biological environment could potentially affect the compound’s solubility and reactivity . Additionally, the presence of other biomolecules could influence the compound’s ability to bind to its targets and induce protein degradation
Biochemical Analysis
Biochemical Properties
Bromo-PEG1-Acid plays a significant role in biochemical reactions, particularly in proteomics research . It interacts with various enzymes, proteins, and other biomolecules. The nature of these interactions is largely dependent on the bromide group, which can participate in nucleophilic substitution reactions .
Molecular Mechanism
At the molecular level, this compound exerts its effects through its bromide group and carboxyl group. The bromide group can participate in nucleophilic substitution reactions, while the carboxyl group can form covalent bonds with proteins and other biomolecules .
Transport and Distribution
The transport and distribution of this compound within cells and tissues are likely influenced by its chemical structure. The hydrophilic PEG spacer may facilitate its distribution in aqueous environments .
Preparation Methods
Synthetic Routes and Reaction Conditions
Bromo-PEG1-Acid can be synthesized through a series of chemical reactions involving the introduction of a bromo group and a carboxyl group to a PEG chain. The typical synthetic route involves the reaction of polyethylene glycol with bromoacetyl bromide in the presence of a base such as triethylamine. The reaction is carried out under anhydrous conditions to prevent hydrolysis of the bromoacetyl bromide .
Industrial Production Methods
In industrial settings, the production of this compound involves similar synthetic routes but on a larger scale. The reaction conditions are optimized to ensure high yield and purity of the final product. The compound is typically purified through recrystallization or chromatography techniques to remove any impurities .
Chemical Reactions Analysis
Types of Reactions
Bromo-PEG1-Acid undergoes various chemical reactions, including:
Nucleophilic Substitution: The bromo group is a good leaving group, making the compound suitable for nucleophilic substitution reactions.
Esterification: The carboxyl group can react with alcohols to form esters.
Common Reagents and Conditions
Nucleophilic Substitution: Common reagents include nucleophiles such as amines or thiols.
Esterification: Reagents include alcohols and a catalyst such as sulfuric acid or p-toluenesulfonic acid.
Major Products Formed
Nucleophilic Substitution: The major products are substituted PEG derivatives where the bromo group is replaced by the nucleophile.
Esterification: The major products are PEG esters.
Scientific Research Applications
Bromo-PEG1-Acid has a wide range of applications in scientific research:
Comparison with Similar Compounds
Similar Compounds
Bromo-PEG2-Acid: Contains an additional PEG unit, providing greater solubility and flexibility.
Bromo-PEG3-Acid: Contains two additional PEG units, further enhancing solubility and flexibility.
Propargyl-PEG1-Acid: Features a propargyl group instead of a bromo group, offering different reactivity.
Uniqueness
Bromo-PEG1-Acid is unique due to its balance of hydrophilicity and reactivity. The single PEG unit provides sufficient solubility without significantly increasing the molecular weight, making it suitable for various applications. Its bromo group offers a versatile site for nucleophilic substitution, while the carboxyl group allows for further functionalization .
Properties
IUPAC Name |
3-(2-bromoethoxy)propanoic acid | |
---|---|---|
Source | PubChem | |
URL | https://pubchem.ncbi.nlm.nih.gov | |
Description | Data deposited in or computed by PubChem | |
InChI |
InChI=1S/C5H9BrO3/c6-2-4-9-3-1-5(7)8/h1-4H2,(H,7,8) | |
Source | PubChem | |
URL | https://pubchem.ncbi.nlm.nih.gov | |
Description | Data deposited in or computed by PubChem | |
InChI Key |
REBYHCQUFPLTTH-UHFFFAOYSA-N | |
Source | PubChem | |
URL | https://pubchem.ncbi.nlm.nih.gov | |
Description | Data deposited in or computed by PubChem | |
Canonical SMILES |
C(COCCBr)C(=O)O | |
Source | PubChem | |
URL | https://pubchem.ncbi.nlm.nih.gov | |
Description | Data deposited in or computed by PubChem | |
Molecular Formula |
C5H9BrO3 | |
Source | PubChem | |
URL | https://pubchem.ncbi.nlm.nih.gov | |
Description | Data deposited in or computed by PubChem | |
Molecular Weight |
197.03 g/mol | |
Source | PubChem | |
URL | https://pubchem.ncbi.nlm.nih.gov | |
Description | Data deposited in or computed by PubChem | |
Retrosynthesis Analysis
AI-Powered Synthesis Planning: Our tool employs the Template_relevance Pistachio, Template_relevance Bkms_metabolic, Template_relevance Pistachio_ringbreaker, Template_relevance Reaxys, Template_relevance Reaxys_biocatalysis model, leveraging a vast database of chemical reactions to predict feasible synthetic routes.
One-Step Synthesis Focus: Specifically designed for one-step synthesis, it provides concise and direct routes for your target compounds, streamlining the synthesis process.
Accurate Predictions: Utilizing the extensive PISTACHIO, BKMS_METABOLIC, PISTACHIO_RINGBREAKER, REAXYS, REAXYS_BIOCATALYSIS database, our tool offers high-accuracy predictions, reflecting the latest in chemical research and data.
Strategy Settings
Precursor scoring | Relevance Heuristic |
---|---|
Min. plausibility | 0.01 |
Model | Template_relevance |
Template Set | Pistachio/Bkms_metabolic/Pistachio_ringbreaker/Reaxys/Reaxys_biocatalysis |
Top-N result to add to graph | 6 |
Feasible Synthetic Routes
Disclaimer and Information on In-Vitro Research Products
Please be aware that all articles and product information presented on BenchChem are intended solely for informational purposes. The products available for purchase on BenchChem are specifically designed for in-vitro studies, which are conducted outside of living organisms. In-vitro studies, derived from the Latin term "in glass," involve experiments performed in controlled laboratory settings using cells or tissues. It is important to note that these products are not categorized as medicines or drugs, and they have not received approval from the FDA for the prevention, treatment, or cure of any medical condition, ailment, or disease. We must emphasize that any form of bodily introduction of these products into humans or animals is strictly prohibited by law. It is essential to adhere to these guidelines to ensure compliance with legal and ethical standards in research and experimentation.