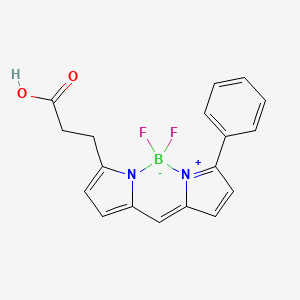
Bodipy R6G
Overview
Description
Bodipy R6G, also known as BDP R6G, is a borondipyrromethene dye . It is tuned to match Rhodamine 6G (R6G) excitation and emission channels . This fluorophore possesses an excellent extinction coefficient and emission quantum yield . It is very photostable, and its excited state has a reasonably long lifetime . Therefore, it can be used for fluorescence polarization assays .
Synthesis Analysis
The synthesis of commercial Bodipy and its derivatives involves processes such as electrochemical halogenation, Suzuki coupling, and ester hydrolysis . Bodipy R6G dye can be incorporated at any position within an oligonucleotide post-synthetically . A primary amine modified oligonucleotide is used to covalently attach with dye via NHS ester chemistry .Molecular Structure Analysis
The molecular formula of Bodipy R6G is C18H15BF2N2O2 . The molecular weight is 340.13 . The structure of Bodipy dyes, including Bodipy R6G, is based on the borondipyrromethene core .Chemical Reactions Analysis
Bodipy R6G has been used for developing fluorescent intracellular tracers . Its conjugates are cell-permeable as long as its carriers are cell-permeable . Bodipy dyes have been turned from highly fluorescent labels into efficient triplet photosensitizers with strong absorption in the visible region .Physical And Chemical Properties Analysis
Bodipy R6G has absorption max at 527 nm and emission max at 547 nm . It is very photostable, and its excited state has a reasonably long lifetime . The photophysical properties and photodegradation of Bodipy derivatives have been studied extensively .Scientific Research Applications
Dual Modality Imaging Agents
Bodipy R6G has been utilized in the development of dual modality imaging agents, combining positron emission tomography (PET) and fluorescence imaging. This integration is particularly valuable for tumor imaging. BODIPY dyes can be converted into radioactive analogues through an 18F-19F isotopic exchange reaction, making them suitable for PET imaging. For example, an integrin-targeting RGD peptide conjugated with [18F]BODIPY® R6G demonstrated notable tumor uptake in the U87MG xenograft model (Liu et al., 2013).
Versatile Fluorophore for Various Applications
BODIPY dyes, including R6G, are recognized for their versatility as fluorophores, offering a broad range of applications in photochemistry and biophotonics. Their structural adaptability allows modulation of spectroscopic properties and photophysical processes, expanding their use in technological and scientific research (Bañuelos, 2016).
Nuclear and Optical Dual Functional Labeling
BODIPY R6G has been applied in the development of nuclear and optical dual functional labeling agents for proteins and peptides. Radioiodinated BODIPY, for instance, has been used in single photon emission computed tomography (SPECT) imaging studies, demonstrating its potential in both basic and clinical research (Ono et al., 2017).
Triplet Excited State Applications
The study of the triplet excited state of Bodipy, including R6G, has significant implications for photodynamic therapy (PDT), photocatalysis, and triplet-triplet annihilation upconversion. Methods for the formation and modulation of the triplet excited state of Bodipy have been explored, indicating its importance in various applications ranging from medicinal to photophysical research (Zhao et al., 2015).
Fluorescent Probes for ROS/RNS and Cancer Diagnosis
BODIPY-based molecules, including R6G, have been developed as fluorescent probes for detecting reactive oxygen/nitrogen species (ROS/RNS) and for early-stage cancer diagnosis. Their unique photophysical properties and chemical stability make them ideal candidates for biomedical applications (Nguyen et al., 2021).
Quantitative Detection of Specific DNA/RNA
BODIPY R6G-based oligonucleotide probes or primers have been utilized for the quantitative detection of specific DNA or RNA molecules. This technique leverages the quenching of BODIPY fluorescence by guanine, providing a direct method for DNA/RNA quantification (Kurata et al., 2001).
Mechanism of Action
Future Directions
Bodipy dyes, including Bodipy R6G, have spectral characteristics that are often superior to those of other dyes . With derivatives that span the visible spectrum, Bodipy dyes are extremely versatile . They are used to generate fluorescent conjugates of various biomolecules . The future of Bodipy dyes lies in their potential for numerous chemical and biophysical applications .
properties
IUPAC Name |
3-(2,2-difluoro-12-phenyl-3-aza-1-azonia-2-boranuidatricyclo[7.3.0.03,7]dodeca-1(12),4,6,8,10-pentaen-4-yl)propanoic acid | |
---|---|---|
Source | PubChem | |
URL | https://pubchem.ncbi.nlm.nih.gov | |
Description | Data deposited in or computed by PubChem | |
InChI |
InChI=1S/C18H15BF2N2O2/c20-19(21)22-14(9-11-18(24)25)6-7-15(22)12-16-8-10-17(23(16)19)13-4-2-1-3-5-13/h1-8,10,12H,9,11H2,(H,24,25) | |
Source | PubChem | |
URL | https://pubchem.ncbi.nlm.nih.gov | |
Description | Data deposited in or computed by PubChem | |
InChI Key |
RFLLOBSXBJAGST-UHFFFAOYSA-N | |
Source | PubChem | |
URL | https://pubchem.ncbi.nlm.nih.gov | |
Description | Data deposited in or computed by PubChem | |
Canonical SMILES |
[B-]1(N2C(=CC=C2CCC(=O)O)C=C3[N+]1=C(C=C3)C4=CC=CC=C4)(F)F | |
Source | PubChem | |
URL | https://pubchem.ncbi.nlm.nih.gov | |
Description | Data deposited in or computed by PubChem | |
Molecular Formula |
C18H15BF2N2O2 | |
Source | PubChem | |
URL | https://pubchem.ncbi.nlm.nih.gov | |
Description | Data deposited in or computed by PubChem | |
Molecular Weight |
340.1 g/mol | |
Source | PubChem | |
URL | https://pubchem.ncbi.nlm.nih.gov | |
Description | Data deposited in or computed by PubChem | |
Product Name |
BDP R6G carboxylic acid |
Retrosynthesis Analysis
AI-Powered Synthesis Planning: Our tool employs the Template_relevance Pistachio, Template_relevance Bkms_metabolic, Template_relevance Pistachio_ringbreaker, Template_relevance Reaxys, Template_relevance Reaxys_biocatalysis model, leveraging a vast database of chemical reactions to predict feasible synthetic routes.
One-Step Synthesis Focus: Specifically designed for one-step synthesis, it provides concise and direct routes for your target compounds, streamlining the synthesis process.
Accurate Predictions: Utilizing the extensive PISTACHIO, BKMS_METABOLIC, PISTACHIO_RINGBREAKER, REAXYS, REAXYS_BIOCATALYSIS database, our tool offers high-accuracy predictions, reflecting the latest in chemical research and data.
Strategy Settings
Precursor scoring | Relevance Heuristic |
---|---|
Min. plausibility | 0.01 |
Model | Template_relevance |
Template Set | Pistachio/Bkms_metabolic/Pistachio_ringbreaker/Reaxys/Reaxys_biocatalysis |
Top-N result to add to graph | 6 |
Feasible Synthetic Routes
Disclaimer and Information on In-Vitro Research Products
Please be aware that all articles and product information presented on BenchChem are intended solely for informational purposes. The products available for purchase on BenchChem are specifically designed for in-vitro studies, which are conducted outside of living organisms. In-vitro studies, derived from the Latin term "in glass," involve experiments performed in controlled laboratory settings using cells or tissues. It is important to note that these products are not categorized as medicines or drugs, and they have not received approval from the FDA for the prevention, treatment, or cure of any medical condition, ailment, or disease. We must emphasize that any form of bodily introduction of these products into humans or animals is strictly prohibited by law. It is essential to adhere to these guidelines to ensure compliance with legal and ethical standards in research and experimentation.